RFF’s Jay Bartlett and Alan Krupnick evaluate hydrogen and CCUS options for the deep decarbonization of steel production, which is the source of 7 percent of global CO₂ emissions.
This article is part of a series in which we expound on a recent report and explore how decarbonized hydrogen compares to other options for reducing carbon emissions—namely, carbon capture, utilization, and storage (CCUS) and electrification with zero-carbon power. By evaluating decarbonized hydrogen against these other two options, we explore when, where, and how hydrogen is likely to be a cost-effective method for reducing CO₂ emissions. Previous blog posts have considered “blue” and “green” hydrogen and how decarbonized hydrogen can reduce emissions from oil refining and ammonia production. Subsequent articles will assess hydrogen for industrial heat and energy storage as well as policy mechanisms to reduce industrial and power sector emissions.
In addition to replacing “gray” or “brown” hydrogen with “blue” or “green” hydrogen in feedstock applications, decarbonized hydrogen could further reduce emissions by displacing natural gas or coal feedstocks. We find that the largest and most promising new opportunity for decarbonized hydrogen feedstock is in iron and steel production, which annually emits nearly 3 billion tons of CO₂ and represents 7 percent of global CO₂ emissions. It is important to note that iron and steel is not the only sector that could benefit from using decarbonized hydrogen instead of high-carbon feedstocks. Decarbonized hydrogen could be used to produce methanol and urea, for instance, but such an application is unlikely in the near term, given that it would require a non-emitting source of carbon (such as direct air capture) together with decarbonized hydrogen. Therefore, we will focus on iron and steel production in this article, evaluating the use of decarbonized hydrogen against CCUS applied to emissions from natural gas or coal to determine which approach is likely to reduce steelmaking emissions more efficiently.
Steel manufacturing consists of primary production, in which iron ore is converted to iron and then processed into steel, and secondary production, in which scrap steel is recycled. Most primary production occurs through the blast furnace–basic oxygen furnace (BF-BOF) route, though a smaller amount of primary steel is produced from direct reduction of iron (DRI) followed by processing in an electric arc furnace (EAF). Most secondary production, which is less energy intensive, occurs in an EAF. Table 1 presents the quantities of primary and secondary steel production by method, both in the United States and globally. Whereas secondary production using EAF represents 66 percent of US steel production, it amounts to only 22 percent of global steel production. In this article, we will explore the BF-BOF and DRI-EAF steelmaking methods and assess options within each pathway for deep decarbonization.
Table 1. US and Global Steel Production, by Method
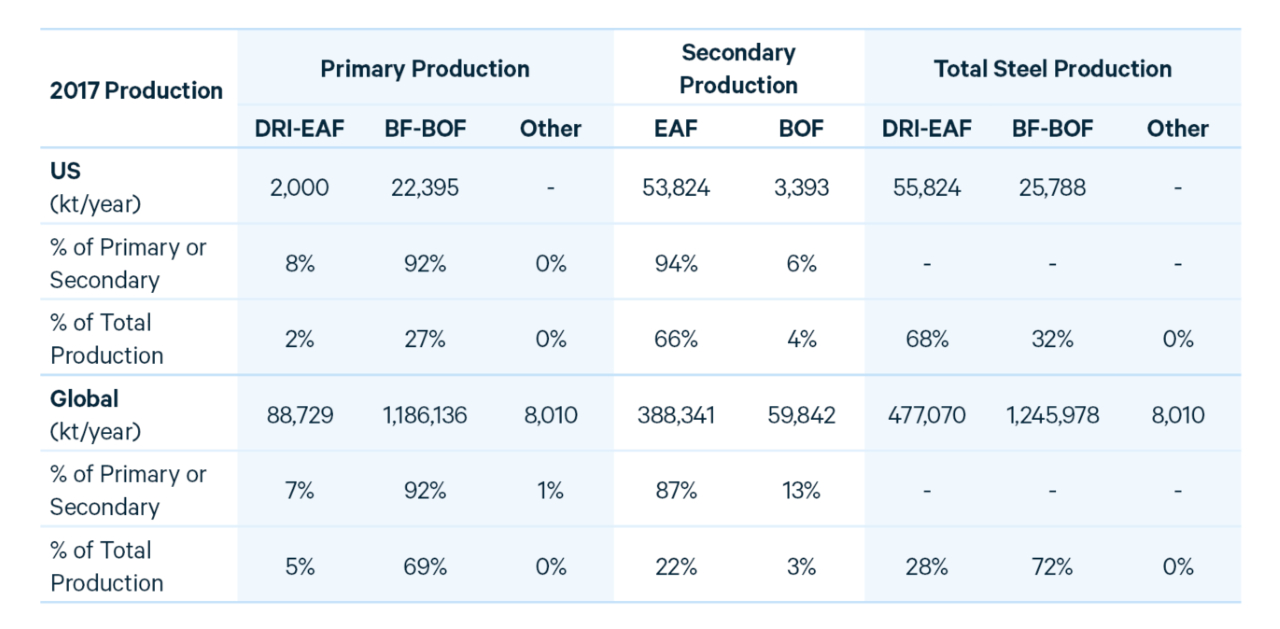
In BF-BOF steelmaking, iron ore, coke (high-carbon coal purged of volatile compounds), and limestone are added to a blast furnace, where the iron ore reacts with carbon monoxide to produce high-carbon crude iron and CO₂. The iron is then transferred to a basic oxygen furnace, where oxygen combines with some of the carbon in the crude iron, resulting in low-carbon steel and additional CO₂. Total CO₂ emissions from the BF-BOF route are high—with an average of 1.73 tons CO₂ per ton of steel—owing to both the process and combustion emissions.
Coal is fundamental to the BF-BOF process, as it provides heat, carbon monoxide, and physical structure for the reaction within the blast furnace. Consequently, complete substitution of coal with a low-carbon source of feedstock and fuel, such as decarbonized hydrogen, is not feasible on a wide scale (although charcoal has been used in some biomass-rich countries, such as Brazil). Hydrogen could reduce the amount of coal consumed in BF-BOF steelmaking, but doing so would only modestly decrease CO₂ emissions.
Given the technical challenges involved in substituting feedstock and fuel, CCUS has the greatest potential globally to decarbonize the BF-BOF process. One study, which reviews three techniques for integrating CCUS in BF-BOF steel production, estimates possible emissions reductions of 47 to 60 percent. To achieve further CO₂ reductions, the HIsarna process—currently in pre-commercial “demonstration” phase—eliminates the emissions-intensive steps of sintering (agglomerating iron ore) and coking. If integrated with CCUS, HIsarna steel production could reduce CO₂ emissions by 80 to 90 percent.
While coal-based DRI-EAF is common in India, a majority of DRI-EAF steelmaking entails the reforming of natural gas to generate a mixture of carbon monoxide and hydrogen—the first step toward producing gray or blue hydrogen. Both carbon monoxide and hydrogen are used to reduce iron ore in a furnace, which then yields solid iron, steam, and CO₂. The iron is then combined with scrap steel and melted in an electric arc furnace, where impurities are removed, to produce molten steel. As shown in Table 2, CO₂ emissions from natural gas–based DRI-EAF steelmaking amount to about half of the emissions from BF-BOF production. The lower CO₂ emissions of natural gas–based DRI-EAF are a consequence of its greater efficiency of feedstock and fuel use as well as the lower carbon content of natural gas relative to coal.
Table 2. Energy and Emission Intensities, by Steelmaking Process
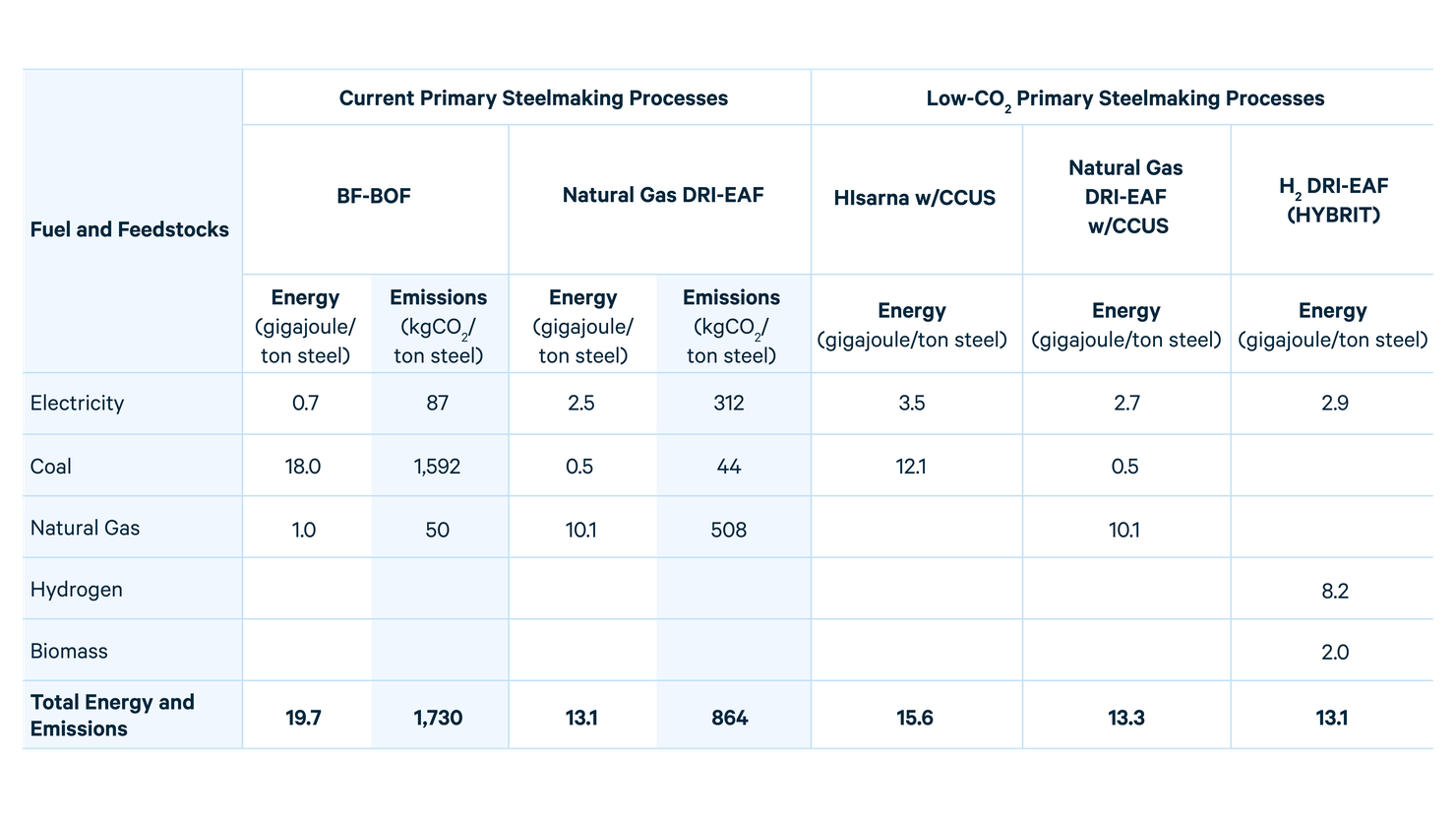
From Table 2, emissions from natural gas–based DRI-EAF could be reduced by a further 35 percent if the electricity comes from zero-carbon sources rather than the US grid. After eliminating electricity emissions, most of the remaining CO₂ comes from the use of natural gas to reduce iron ore, which sets up two pathways for the deep decarbonization of DRI-EAF steelmaking. First, CCUS could be applied to the DRI reactor, capturing CO₂ from the reduction of iron ore. This technology is already in commercial operation in the United Arab Emirates. Second, feedstock for the direct reduction of iron could be decarbonized by substituting pure hydrogen in place of natural gas, a technology proposed by the HYBRIT project in Sweden. Currently in pre-demonstration “pilot” phase, HYBRIT plans to use zero-carbon hydrogen along with renewable power and sustainable biomass to produce steel with minimal emissions.
With our interest in hydrogen, we also would like to determine which type of decarbonized hydrogen—blue or green—might be used in hydrogen-based DRI-EAF steelmaking. From our previous article on decarbonized hydrogen in existing feedstock applications, blue hydrogen is less expensive than green hydrogen in the near term. However, because the DRI process allows for CCUS, first we must evaluate blue hydrogen–based DRI-EAF (i.e., capturing CO₂ before use in DRI) against natural gas–based DRI-EAF with CCUS (i.e., capturing CO₂ after use in DRI). From comparing the two processes, natural gas–based DRI-EAF with CCUS is likely to be more cost effective than blue hydrogen–based DRI-EAF. In contrast, green hydrogen has a production process that is decoupled from natural gas, making green hydrogen the probable (albeit long–term) source for decarbonized hydrogen in steel production.
Looking across the BF-BOF and DRI-EAF steelmaking routes, three pathways—HIsarna with CCUS, natural gas–based DRI-EAF with CCUS, and green hydrogen–based DRI-EAF—all have the potential to cost-effectively reduce emissions by 80 percent or more from the predominant BF-BOF process. Of course, with further research and development, other methods may prove promising as well.
How does the cost of green hydrogen–based DRI-EAF compare with the costs of HIsarna with CCUS and natural gas–based DRI-EAF with CCUS? The International Energy Agency finds that a price of $0.70–$2.00 per kg H₂ is needed for green hydrogen–based DRI-EAF to produce steel at equal cost to natural gas–based DRI-EAF with CCUS. The wide range in hydrogen breakeven prices is a consequence of the large variation in natural gas prices; regions with low natural gas prices (and CO₂ storage) would find natural gas–based DRI-EAF with CCUS particularly competitive. To make green hydrogen–based DRI-EAF competitive with HIsarna and 90 percent CCUS, the Hydrogen Council estimates a breakeven price range of $1.20–$1.60 per kg H₂. The midpoint of these two ranges, about $1.40 per kg H₂, is potentially achievable for the delivered cost of green hydrogen over the long term. But, as we discussed in a previous blog post, production, storage, and transportation costs will need to decline substantially first.
In the near to medium term, emissions from steel production could be reduced by increasing the efficiency and lowering the carbon content of fuel and feedstock. More specifically, raising the proportion of recycled steel and transitioning from coal to natural gas are worthwhile decarbonization approaches in this context. Electrification of heat (e.g., the electric arc furnace) has similar potential, because while average US grid power currently entails high CO₂ emissions, renewable and nuclear power would allow for zero-carbon electric heat. However, to achieve deep decarbonization of steelmaking, feedstock emissions must also be minimized, whether through CCUS or decarbonized hydrogen. Green hydrogen likely has the longest path before it becomes competitive, but if combined with zero-carbon power, green hydrogen has the advantage of enabling steel production with negligible emissions.

Read more articles from the “Potential of Hydrogen for Decarbonization” blog series:
- “Evaluating Low-Carbon “Blue” Hydrogen Against End-Use CCUS” (January 11, 2021)
- “The Potential of Hydrogen for Decarbonization: Evaluating Zero-Carbon 'Green' Hydrogen Against Renewable and Nuclear Power” (January 20, 2021)
- “The Potential of Hydrogen for Decarbonization: Reducing Emissions in Oil Refining and Ammonia Production” (February 4, 2021)
- “The Right Policies Can Incentivize Cleaner “Blue” Hydrogen” (August 27, 2021)
- “Effective Incentives for Hydrogen Production, with Long- and Near-Term Climate Benefits” (November 15, 2021)