Price's Landfill occupies some 22 acres about 5 miles west of Atlantic City, New Jersey. Once a sand and gravel quarry, the pit was excavated to within 2 feet of the water table in 1968 and people from the surrounding area began to dump trash into it with the permission of the owner, Charles Price.
In 1969 Price began commercial landfill operations, and in 1970 he applied to the New Jersey Department of Environmental Protection (NJDEP) for a license to run a sanitary landfill; the application specifically excluded "Chemicals (Liquid or Solid)." The department issued a certificate authorizing operation of a solid waste disposal facility.
The New Jersey State Sanitary Code requires every landfill operator to submit a detailed sanitary design so the NJDEP may determine whether planned operations pose a threat to health or the environment. Price's plan—not submitted until late 1971—did not mention chemicals, despite the fact that the landfill already was beginning to fill with chemical wastes.
Only in 1972 did Price seek authorization to accept and dispose of liquid and chemical wastes. In its response, the NJDEP granted him a certificate, subject to the condition that "no liquid or soluble industrial wastes, petrochemicals, waste oils, sewage sludge, or septic tank wastes shall be received for disposal at this site." But Price continued to accept significant quantities of chemical and liquid wastes. Minimal precautions were taken: wastes often were poured into the landfill from an open spigot on a tank truck, and drums of chemicals were buried under piles of refuse.
In July 1972, the NJDEP inspected the landfill, cited Price for accepting chemical wastes, and formally advised him of the violation. Nonetheless, he continued to handle chemical wastes until November 1972. After that date, no chemical wastes were disposed of at the landfill, although it continued in operation. In 1976 Price terminated the landfill operation and covered the site with fill material. It has not been used since.
During the period from May 1971 to November 1972, Price accepted some 9 million gallons of the following toxic and flammable chemical and liquid wastes, either in drums or directly into the ground: acetone, acids (glycolic, nitric, and sulfuric) and spent acid wastes, acryloid, acryloid monomer and poly acryloid, caulking and spent caulking solvent, caustics and spent caustic wastes, cesspool waste, chemical resins and other waste chemicals, chloroform, cleaning solvents, ether and spent ether wastes, ethyl acetate, ethylene dichloride, fatty acids, glue wastes, grease and spent grease solvents, heptane, hexane, inks and waste ink residues, isopropanol, isopropyl alcohol, isopropyl ether, lacquer thinner, manganese dioxide, methanol, methyl ethyl ketone, methyl isobutyl ketone, methyl vinyl ketone, miscellaneous chemical laboratory wastes, mineral spirits, oil and waste oil products (No. 6 waste oil), paint, paint sludge, paint thinner and spent paint wastes, perfume wastes, phenols, phenolics and phenolic solvents, resins, septic waste and sludge, still bottoms, styrene and styrene wastes, tar, titanium wastes, xylene, and xylol. And this is only a partial list; there were many more.
Extensive water level measurements show that the hydraulic gradient near Price's Landfill slopes generally east and east-northeast from the landfill. The groundwater in the area, and contaminants in that groundwater, therefore tend to flow east and east-northeast. The landfill sits roughly 1 mile west of the sites from which the Atlantic City Municipal Water Authority pumps most of its drinking water. A leachate plume has been defined near the landfill, although its precise contours have not been determined.
No isolated incident
Price's Landfill is not an aberration. According to the U.S. Water Resources Council, "Every region in the country experiences groundwater pollution problems, both point and nonpoint. . . . Their widespread nature supports concern with degradation of groundwater supplies throughout the Nation." The Congressional Research Service identifies more than 100 incidents in which 1,363 wells were closed.The Council on Environmental Quality claims, on the basis of evidence gathered from numerous case studies across the nation, that "hundreds of wells supplying drinking water to millions of people have been closed because of toxic organic chemicals in high concentrations. . . ."
Moreover, it is impossible to know whether the available data reflect the entire range of water quality problems. In almost every case studied to date, only a few of several hundred possible compounds actually were tested for, and then only after contamination was suspected. In many of the cases, well water was found to contain concentrations above—and often several orders of magnitude higher than—those commonly encountered in drinking water drawn from badly contaminated surface water sources. Even at extremely low concentrations, many toxic organic chemicals pose serious, irreversible, health risks.
Among the principal sources of groundwater contamination are waste disposal landfills and impoundments, accidental spills, and abandoned oil and gas wells. In the fall of 1983, the U.S. Environmental Protection Agency warned of an additional and ubiquitous source—underground gasoline storage tanks at thousands of service stations across the country. But most groundwater contamination can be traced to chemicals leaching into the aquifer from poorly constructed and managed industrial or municipal landfills, surface impoundments, or outright illegal dumps. Contamination from such sources often has been in process for years—sometimes for decades—and most contamination incidents are discovered only after a drinking water source has been affected.
By the time suspected aquifer contamination is verified in samples drawn from drinking water wells, the problem may be irreversible. Stricter regulation of the disposal of potential contaminants in other environmental media, particularly air and surface waters, and the consequent rising cost of such disposal, is likely to increase the flow of wastes to land disposal. But groundwater use has grown faster, over the past twenty-five years, than population: from 12.4 trillion gallons per year in 1950 to almost 30 trillion gallons per year in 1975. It is thus likely that a growing portion of the population is being exposed to contaminants in groundwater. Because cleanup is a formidable task, often prohibitively expensive or technically infeasible, preventive measures may be the only effective means of protecting groundwater resources.
As researchers, we are particularly concerned with assessing the benefits (costs avoided) of controlling groundwater contamination. But benefit-cost analyses of groundwater contamination require quantification of several links between sources and receptors. We must know the location and strength of actual or potential sources of contamination. We must be able to model the spread of the contaminant plume in the aquifer. We must know the numbers of persons exposed to contaminated groundwater and the extent and timing of their exposures. We must know the "dose-response relationship"—the nature and extent of health effects on the population at risk. And finally, we need a way of converting health effects into monetary, or dollar, values.
This is a very tall order, and we are far from being able to quantify these links with precision. In each case, we need substantially improved methods and data. For the purposes of this article, therefore, we concentrate on a few less ambiguous aspects of the problem.
Remedial and decontamination measures
In contemplating the future of hazardous waste disposal and the protection of groundwater, the main task for public policy is providing appropriate institutions and incentives for environmental protection. But for a case like Price's Landfill, a fait accompli, the choices are narrow: either to bear the risk or take remedial action.
Groundwater contamination can be contained using various techniques. Direct methods are designed to prevent contaminated fluids from an impoundment or leachates from a landfill from coming into contact with uncontaminated groundwater. These include installation of impermeable material below the site (applicable chiefly to new sites), collection and treatment of contaminated groundwater and recharge to the aquifer or to a surface stream, construction of a groundwater cutoff wall, and capping surface of an impoundment or a landfill to prevent leaching induced by precipitation. Indirect methods involve developing or providing substitute sources of water or treating contaminated water just before use.
In almost all actual groundwater contamination episodes, only the least expensive of the direct methods have been employed, such as covering the site to prevent leaching. Cleaning an aquifer is an extremely costly, complicated, and lengthy undertaking, with success uncertain, and authorities usually resort to indirect methods.
Here we consider two alternative remedial schemes for cleaning the Cohansey aquifer, the principal source of Atlantic City's water supply. Both involve indirect cleaning operations: pumping contaminated water, treating it, and then recharging the aquifer with the treated water. The two schemes are:
- Cessation of pumping at the shallow Atlantic City Municipal Authority wells; pumping 30 million gallons per month from the upper Cohansey at three specific locations in the well field; and decontaminating the water and injecting it at three locations near the landfill.
- The first two steps are identical to those in the first alternative, with the addition of three wells downgradient, but near the landfill. The treated water is reinjected at four locations between the landfill and the well field at a rate of 8 million gallons per month.
For both schemes, we assume that contamination of the Cohansey aquifer by leachate from the landfill has been in progress for ten years when remedial measures are initiated and that leachate infiltration is halted at that time. Both alternatives involve flushing pollutants from the aquifer with the help of a hydraulic gradient created by pumping and reinjection.
Analyses of the first alternative indicate that, after ten additional years, the contaminant plume has moved eastward and been halted just short of the production well field. At the cost of pumping, treating and injecting 30 million gallons per month for ten years, we still are far from effective decontamination of the aquifer.
The second scheme attempts removal of the contaminant at the landfill itself. Here only 8 million gallons per month (out of the total 30) are reinjected at points downstream, with the rest disposed of outside the aquifer through sales to industrial users or discharges into streams. This seems much more effective. The leading contour of the contaminant plume remains stationary during the first five years and contracts during the second five years. This is achieved by pumping the mass of contamination near its source, and then lining the plume and diluting it by the injection of treated water downstream from the landfill. But even under this second alternative, which is about as costly as the first, effective cleanup is achieved only after twenty-five years of continuous operation.
The cost estimates for cleanup techniques presented in table 1 are "average-site costs;" actual costs will depend on the situation at the particular site. Within each method category, one or more of the listed activities may be required in any given case. Some actions are complementary, such as those listed under groundwater flow control and plume management. Groundwater flow control must be accompanied by pumping to prevent overflow conditions at the landfill.
If contaminated water removed is reinjected or discharged to a surface water conduit, it must be treated to some extent beforehand (in fact, it is difficult to envisage a situation where it would not). That cost of treatment does not appear in table 1, and must be added to the cost of remedial actions. A recent study estimates the annual cost of such treatment at $500,000. The Cohansey aquifer would require about twenty years of treatment and reinjection. At a discount rate of 10 percent, the present value cost of treatment would be some $4.25 million.
Table 1. Summary of Estimated Average Costs and Characteristics of Direct Remedial Methods
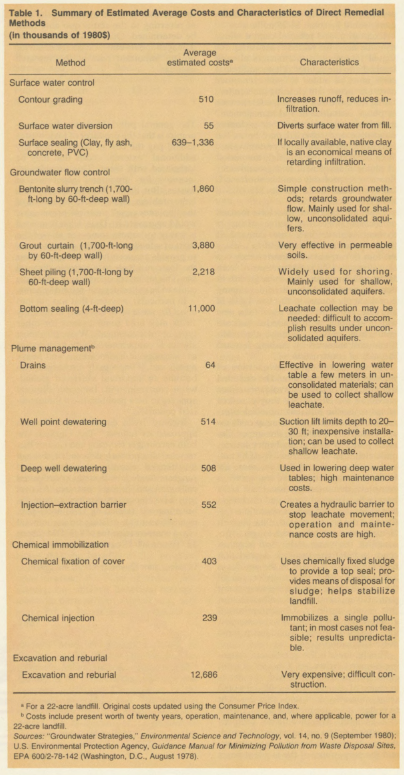
Excavation and reburial cannot eliminate the need for remedial measures designed to remove contaminants already leached into the aquifer. Those additional costs must be added to whatever other costs are incurred in cleaning the aquifer. Using the table as a rough guide, the cost of plume containment and management, including water treatment but excluding excavation and reburial, may be in the range of $5 to $8 million in present value. With excavation and reburial, the period of plume management and groundwater flow control probably could be shortened, but total costs could rise to $15 to $18 million in present value.
Other unofficial estimates have focused on securing alternative sources of supply to meet Atlantic City's water demand. If that requires a new well field to replace the four threatened wells (one already was shut down in 1981), estimates vary from $6.5 to $9.3 million. But since growth in demand would have required developing a new supply source in the late 1980s in any case, only part of the cost of the new well field should be attributed to the contamination episode, namely, the additional cost incurred by pushing forward the date of construction.
All this leaves open the question of whether cleanup costs should be excluded from the cost of damage avoidance, since providing an alternative supply source seems to be the efficient remedial alternative. By not including cleanup costs we are effectively scrapping the upper Cohansey aquifer as a groundwater source, until such time as it cleanses itself by natural processes. Recall that the more effective cleanup scheme did not result in complete decontamination even after twenty-five years of simulated discharge, treatment, and reinjection operations (although it did halt the spread of the plume). Natural processes take much longer to accomplish the same task. And acquiescence in indefinite resource degradation is rational only if water in the aquifer is a free good with zero scarcity rent in all the periods along an optimal management path. Given trends in groundwater use, this seems unlikely. Uncontaminated groundwater almost surely has some positive present worth that would justify some decontamination steps.
Finally, there is the question of assigning property rights. As a matter of principle, and in connection with the determination of damages and liability, it would seem imperative to assume that the right to pollute does not reside with the owner of the landfill. Whether or not compensation for damages can be achieved through the courts, remedial measures are taken, and the perpetrator of the incident bears the full cost of those measures, the cost of reverting to the status quo ante must be determined. Questions of liability for damages and its effect on future behavior are an important part of the research agenda.
Preventing future episodes
The general principle drawn from economics is that the producer of toxic wastes should pay the cost of disposal plus any additional "external" costs that may be associated with them. This provides an incentive for "optimum" generation of wastes. But illegal disposal already is a large problem, and effective imposition of the "polluter pays" principle presumably would aggravate it. Thus, what is needed is a way to impose some—or all—of the costs of safe disposal on the generator and to provide an incentive to actually deliver the toxic residuals to a suitable disposal site. This invites consideration of what have come to be called deposit-refund systems.
In particular, a system that was put into effect for waste oil in Germany in the early 1970s may hold some lessons for other types of toxic chemicals. Waste oil almost always is contaminated with such toxins as carcinogenic hydrocarbons and heavy metals and, if improperly discharged, can become an important source of groundwater contamination. Improper disposal appears to be a problem in all industrialized countries.
Richard Irwin describes the German approach as follows: The German law both encourages recycling and ensures that recycled oil is properly disposed of through a system of reporting requirements and program-funded, nationally coordinated collection and disposal contracts. All persons who import or produce certain lubricating oils (including rerefiners) pay, in addition to an existing tax on mineral oils, a compensation fee of 9.00 DM (about $3.60) per 100 kilograms of product. This money goes into a special fund reserved for the support of the disposal of used oils by controlled burning or recycling, the two methods deemed safe from environmental and public health viewpoints. The fund also supports the program-related administrative expenses of the Federal Office for Trade and Industry.
The enterprises that contract with the Federal Republic are reimbursed for the costs of collection, transportation, and disposal to the extent that these are not covered by the revenues from selling rerefined products. These reimbursements are made at standard rates subject to some flexibility.
In return, the disposal firm has obligations, described by Irwin: The disposal firms' contracts obligate them to (1) pick up all amounts of used oils over 200 liters in the district assigned to them; (2) do so at no charge to the user unless the oils contain more than 10 percent foreign matter; (3) provide suitable containers for lesser amounts so they can be collected later; (4) keep records of their costs, making their books and other relevant information available to the Federal Office or to appointed auditors; (5) file their applications for payments monthly; (6) install equipment specified by the Federal Office for purposes of checking their output; (7) give notice of any rerefined products shipped to other member nations the European Community and return any payments received for producing these products (this requirement is necessary to avoid favoring German rerefiners in violation of the Treaty of Rome); and (8) give receipts for used oils collected which contain more than 10 percent foreign matters.
The German system is a deposit—refund, system where the refund is free disposal of the generators' waste oil. Irwin sums up: The German program is designed to minimize program costs while encouraging recycling. By placing the financial burden of administrative costs and compensation payments on those using the oil, costs to the government are kept to a minimum. Further, since only lubricating oils subject to the existing mineral oil tax are also subject to the disposal fund compensation fee, the paperwork, procedures, and personnel for levying the fee are integrated almost completely with the collection of the mineral oil tax, resulting in substantial administrative cost savings. Finally, the compensation payments encourage collection and recycling of oils that otherwise could not be handled economically in the private sector.
Waste oil may be much more amenable to a deposit-refund system than most toxic wastes. Still, the functioning of the German law deserves careful study, and it would seem to be worth examining other types of toxic waste (contaminated industrial acids and plating plant wastes,for example) to see if an effective deposit-refund system could be devised.
Research needs
In an expanded version of this article we consider three kinds of issues that seem particularly troublesome. The first might be called the need for casting ground-water solute transport modeling in a suitable decision-theoretic framework. Each of the existing models requires knowledge of many geohydrological parameters and, of course, of the solute source terms before ambient concentrations (and thus exposures) can be predicted. But information on those parameters, and on the source terms, only can be gotten at substantial cost—in drilling and sampling. Since costs always will be constrained, there is an obvious question, What is the best way to allocate any such sum among drilling, sampling, and modeling? "Best" should mean something like "giving the most precise exposure forecast."
The second area of needed research is valuation of the particular kind of risk posed to human health by exposure to groundwater contamination. A substantial spread exists in the values individuals attach to risks to life, a range open to at least two interpretations: Individuals may value different kinds of risks to life very differently; or the descriptive realism of expected utility theory is questionable. Both interpretations warrant close invesigation, but the latter is particularly important because it is risk perceptions that matter to individuals and determine individual valuation of risk. Expected utility theory grew up around the study of insurance and gambling behavior, both of which have an actuarial basis for risk asessrnent. Perceptions of the risks of major hazardous episodes—by definition in events—almost certainly work very differently.
Third is the general area of institutional arrangements for reducing risks from major episodes, and for allocating the burdens of those remaining risks society chooses to bear. Our brief discussion of the German waste oil program only suggests how much remains to be done here. In that case, there was an (implicit) decision that the benefits of the program outweighed the considerable transaction costs involved. For other hazardous substances, can similarly beneficial arrangements be devised? Put another way, how many Price's Landfills can the nation afford?

Authors Mark F. Sharefkin, Allen V. Kneese, and Mordechai Shechter are, respectively, fellow and seniorfellow in RFFs Quality of the Environment Division and associate professor of economics at the University of Haifa, Israel. This article is adapted from "Impacts, Costs, and Techniques for Mitigation of Contaminated Groundwater." This longer paper, which emphasizes benefit-cost considerations, is available as RFF reprint 210.