Reducing greenhouse gas emissions will limit further increases in global temperature and reduce the negative effects of climate change. An important challenge is to find the most efficient balance in abating both methane and carbon dioxide.
Methane is a significantly stronger greenhouse gas than carbon dioxide. An important policy question is whether to direct more efforts toward reducing methane emissions or toward mitigating carbon dioxide emissions.
One kilogram of methane in the atmosphere is roughly 80 times more potent than a kilogram of carbon dioxide. Furthermore, methane adds water vapor to the stratosphere and ozone to the troposphere, so the resulting warming effect is roughly 120 times stronger than that of carbon dioxide. However, the atmospheric lifetime of methane is shorter (approximately a decade), while the lifetime of carbon dioxide in the atmosphere is much longer (Figure 1). In fact, after 100 years, around 40 percent of emitted carbon dioxide remains in the atmosphere. After 1,000 years, some 20 percent remains. The exact shares depend on the size of the cumulative historic emissions: the more we have emitted in the past, the higher the share of a pulse emission that will remain over time.
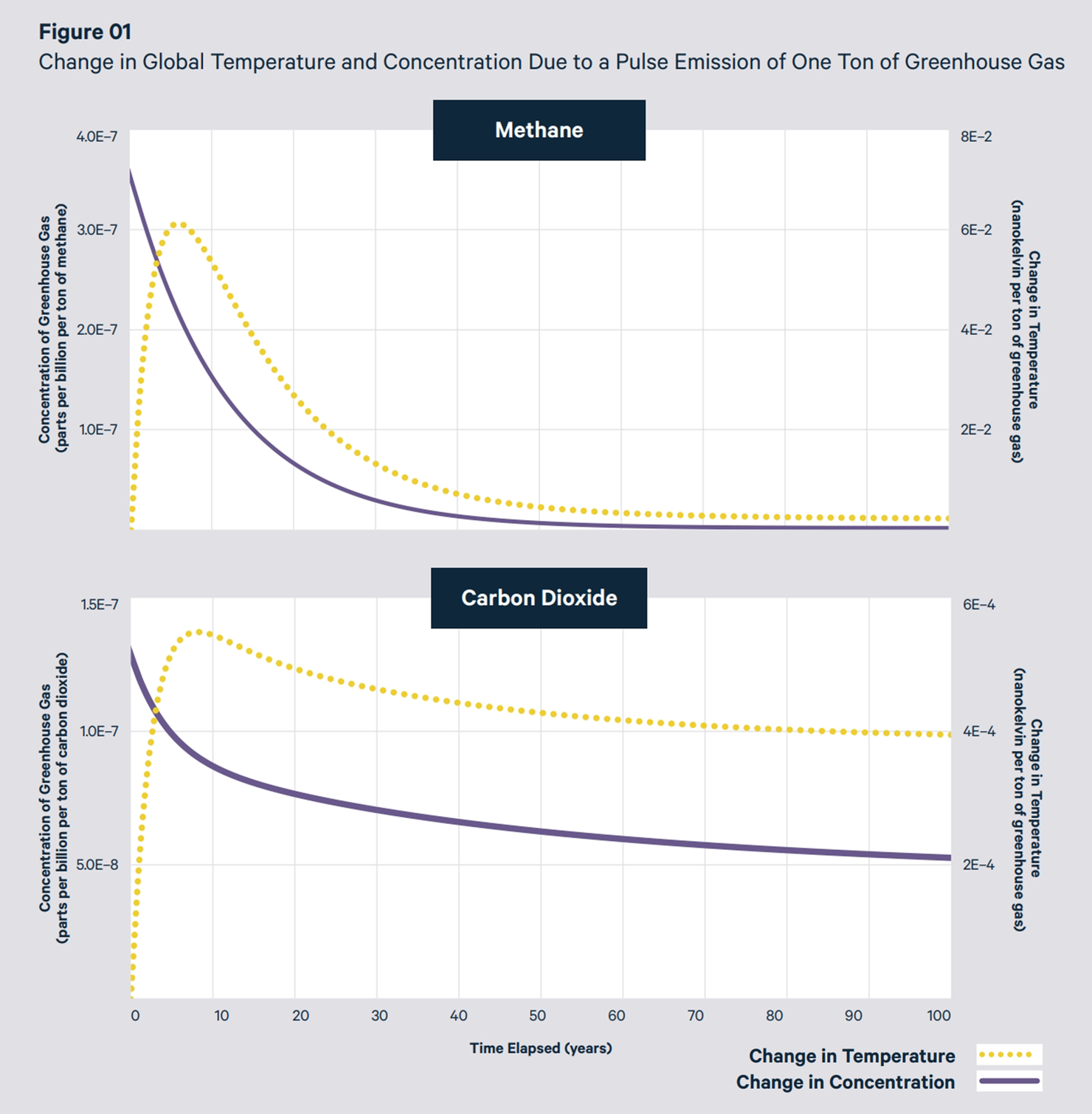
For climate policy to be efficient, the warming effects of carbon dioxide and methane have to be compared—or, more specifically, made comparable—on a common scale. This comparison is difficult, but the United Nations Framework Convention on Climate Change and others typically tackle it by using the so-called global warming potential (GWP) metric.
The GWP shows the cumulative warming effect of an emission of 1 kilogram (kg) of methane over a specific time horizon, typically 100 years, and compares that value with the warming effect of an emission of 1 kg of carbon dioxide over that same horizon. Notably, effects in each year of the horizon are treated equally, and effects beyond the horizon are ignored. The GWP for methane evaluated after 100 years (i.e., GWP100) is about 27.
Critiques have been directed against this method of comparing greenhouse gases. One critique focuses on the fact that the GWP fails to consider certain key economic features. For example, the GWP does not consider discounting nor likely nonlinearities in damages.
Another critique points out that the GWP fails to portray important dynamic features. For instance, stabilizing the global temperature requires emissions of carbon dioxide to reach net zero, but stabilizing the temperature would be possible even if methane emissions continued at a constant rate in perpetuity. The GWP metric fails to convey this dynamic feature.
The trade-off between carbon dioxide and methane entails several dimensions, and opinions vary on the nature of the trade-off. Some analysts argue that GWP100 overvalues the climate impacts of methane; others say that GWP100 undervalues them; and yet others argue that the GWP is irrelevant or misleading, that we can have perpetual emissions of methane, and that virtually all abatement efforts should be directed toward carbon dioxide and other long-lived greenhouse gases.
In this article, we’ll first delve deeper into the dynamic features of methane and carbon dioxide. Then, we’ll further explore the arguments about how much effort should be put into reducing methane in relation to carbon dioxide. Finally, we’ll present recent estimates of the social costs of methane and carbon dioxide. The ratio of the social cost of methane to the social cost of carbon can serve as a possible metric to guide the trade-offs in abatement efforts between these two important greenhouse gases. The approaches and problems discussed here are relevant not only for methane, but also for other climate forcers such as black carbon and contrails.
Basic Properties of Methane and Carbon Dioxide
The key reason why no single method exists to compare methane and carbon dioxide is that their respective lifetimes in the atmosphere are different.
Figure 1 shows the concentration and temperature responses associated with one unit emission of each respective greenhouse gas. The concentration immediately starts to fall after the pulse emission. The temperature response, in contrast, first lags behind the concentration response, then lingers for a longer period of time, which reflects the inertia of the climate system.
When evaluating the GWP of methane, a time horizon must be set over the cumulative warming from the methane emissions (known as the radiative forcing). According to the latest assessment by the Intergovernmental Panel on Climate Change, the GWP20 (i.e., the GWP over a time horizon of 20 years) is 80, GWP100 is 27, and GWP500 is just 7. That is, over longer time horizons, carbon dioxide becomes more important and methane relatively less so. Crucially, no value for the time horizon is objectively correct—subjective choices, laden with value judgments, must be made.
Comparing Sustained Emissions Rather than Emission Pulses
Let us now switch from analyzing emission pulses to constant rates of emissions. What happens to atmospheric concentrations of these greenhouse gases and the average global temperature under sustained emissions?
Figure 2 shows the increase in average global surface temperature with sustained emissions of 1 ton of methane per year for 500 years, compared to sustained emissions of 27 tons of carbon dioxide per year. The rationale for this comparison is that, according to the GWP100 metric, 1 ton of methane per year over 100 years warms the climate by about as much as 27 tons of carbon dioxide per year over the same period. These emissions are equivalent in terms of their GWP, but we see that the dynamics of the temperature responses are very different.
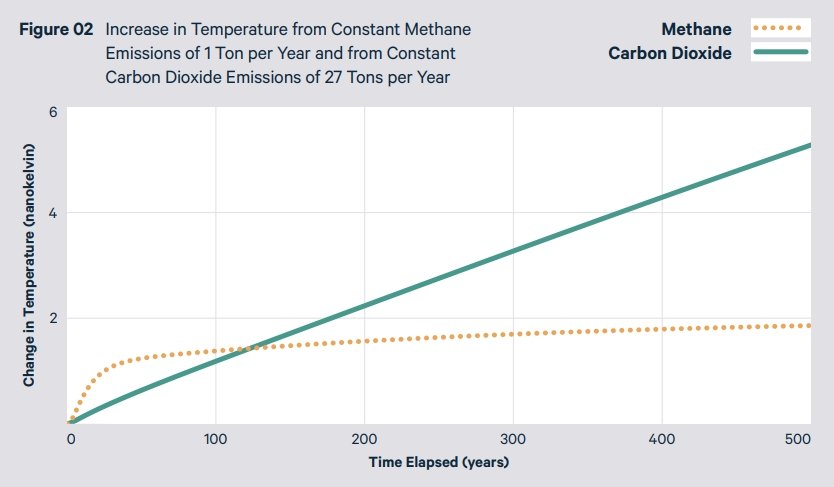
For methane, we see a fast initial response, but after a few decades, the temperature begins to plateau, eventually stabilizing over the long term, even as methane emissions continue at the same rate. The temperature increase associated with this sustained, constant level of methane emissions continues slowly for many hundreds of years before stabilizing due to the thermal inertia of the oceans and feedbacks from the carbon cycle.
For carbon dioxide, on the other hand, we get a roughly constant rate of temperature increase. After about 100 years, the temperature increases to the same level as in the scenario with methane (which might be expected, given that 27 kg of carbon dioxide should be equivalent to 1 kg of methane on a 100-year time horizon, as shown by Azar and Johansson in their 2012 paper). However, whereas the temperature continues to increase with sustained carbon dioxide emissions, that’s not the case with sustained methane emissions.
Constant methane emissions eventually lead to a stable but elevated temperature because methane has a short lifetime in the atmosphere. After a few decades of sustained methane emissions, the added emissions are balanced out by the breakdown (oxidation) of methane. In essence, the more methane we have in the atmosphere (due to our emissions), the more (in absolute terms) is broken down (removed) from the atmosphere through chemical reactions, primarily with the hydroxyl radical.
Constant carbon dioxide emissions would lead to a sustained increase in global temperature because carbon dioxide has a long lifetime and accumulates in the atmosphere. Research has well established that each ton of carbon dioxide emissions yields a near-permanent elevated temperature (Figure 1), and a constant rate of carbon dioxide emissions yields a nearly constant rate of global temperature increase (Figure 2).
Thus, an important asymmetry emerges: constant carbon dioxide emissions yield a sustained rate of increase in the global temperature, whereas constant methane emissions lead to a near-stable temperature increase.
An important conclusion ensues for the debate concerning methane vs carbon dioxide emissions: To stabilize global temperature, emissions of carbon dioxide must eventually drop to zero. Not so for methane, for which constant emissions can be compatible with a stable global temperature.
Policy Positions on Methane vs Carbon Dioxide: Two Contrasting Schools of Thought
These observations have given rise to two fundamentally different positions in the debate over controlling emissions of methane vs emissions of carbon dioxide.
Policy Position 1: Give Methane a Higher Value
Some propose that we need to do much more to limit methane emissions, i.e., more than the warming potential suggested by GWP100. Related arguments typically focus on near-term consequences, such as the risk of passing tipping points in the short term or the need to quickly reduce the current rate of warming. Those who take this position tend to argue in favor of using GWP20 and suggest, for example, that mitigation efforts should focus on reducing methane emissions from shale gas, cattle, or agriculture.
The fundamental problem with this approach, however, is that choosing a 20-year time horizon implies a neglect of the warming impact from year 20 onward, as if what happens in the longer term would not matter.
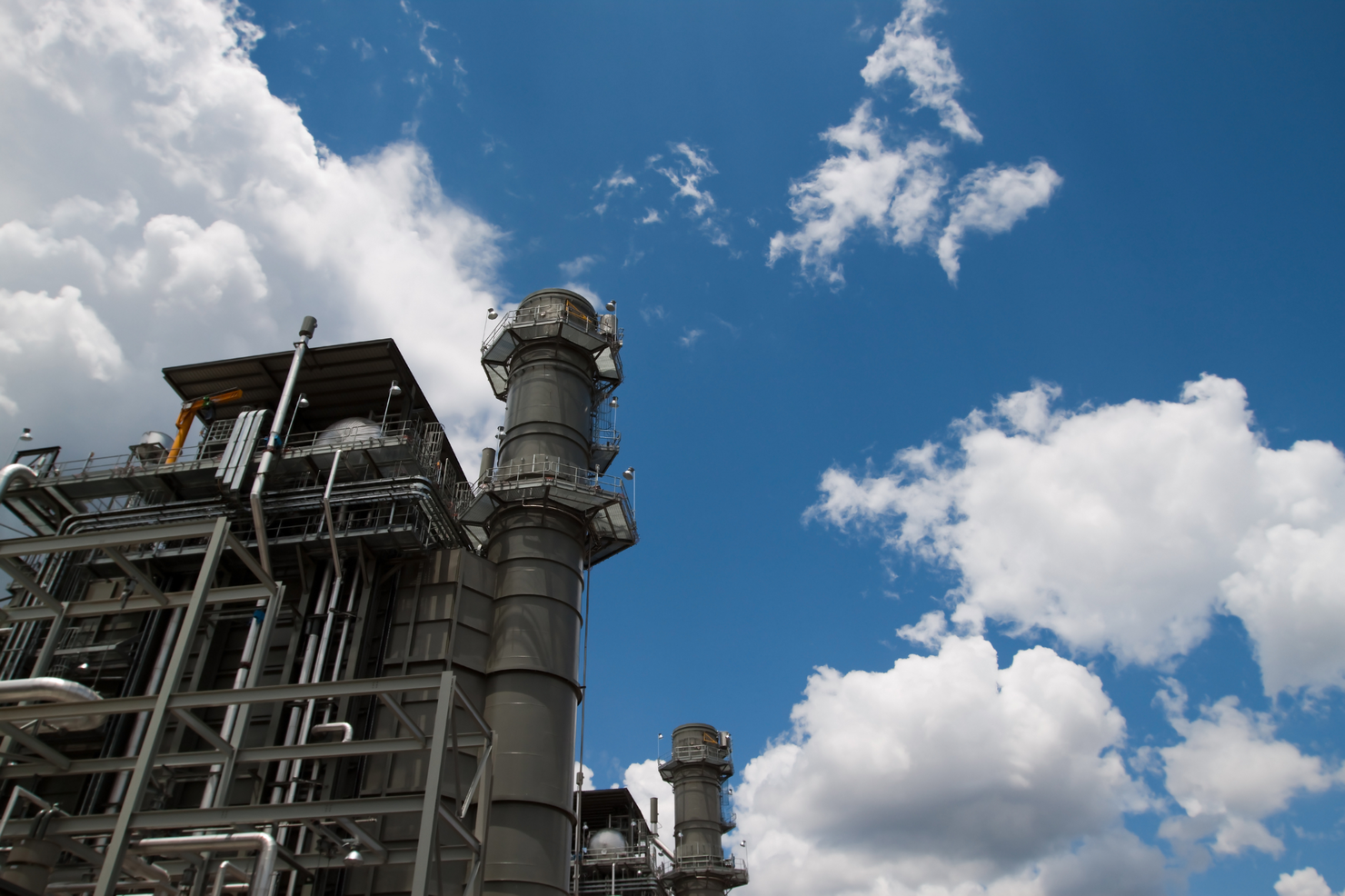
An argument sometimes raised in defense of this position is that the 20-year time horizon should be used only when comparing the impacts of the two greenhouse gases, and that different time horizons should be used for different purposes. But this argument leads to considerable problems of inconsistency. Why should we take a long-term perspective when thinking about how carbon dioxide affects climate change, but consider a short time horizon when comparing greenhouse gases?
Policy Position 2: Methane Is Not (So) Important for Climate Stabilization
A second school of thought argues that GWP100 gives an excessively high value for methane. This argument builds on the dynamic factors mentioned above, that sustained (constant) emissions eventually result in a stable temperature response. The most vulgar version of this argument says that we do not need to reduce methane emissions at all, because constant methane emissions do not produce additional warming above the equilibrium temperature.
However, this argument is irrelevant. The fact remains that every time we emit methane, the global temperature becomes higher than it would have been otherwise.
If the current level of methane emissions is kept constant, then the global temperature eventually would increase by about 1°C above the preindustrial level, in addition to the contribution from carbon dioxide. This increase in temperature would make it essentially impossible to achieve the Paris Agreement target of limiting the increase in global temperature to 1.5–2.0°C.
The trade-off between carbon dioxide and methane entails several dimensions, and opinions vary on the nature of the trade-off.
Furthermore, whereas reducing carbon dioxide emissions only implies a reduction in the rate of increase in global temperature (which is why carbon dioxide emissions eventually must drop to net zero), reducing methane emissions will in fact reduce the global temperature (in terms of the contribution from methane emissions alone to the global temperature response). This reduction in global temperature will in turn reduce the pressure to abate carbon dioxide—and reduce the associated abatement cost for a given level of climate stabilization.
In fact, reducing methane emissions will help achieve climate targets. A balance thus needs to be attained between methane and carbon dioxide. For that purpose, we turn now to economic metrics.
Social Costs of Methane and Carbon Dioxide
An alternative approach to comparing the relative warming impacts of methane and carbon dioxide is to compare the marginal economic damage of emissions of these two gases. The common terminology that describes this economic damage is the “social cost of methane” and the “social cost of carbon dioxide.” (Often with the latter, people skip the “dioxide,” saying instead, “social cost of carbon.”) The ratio between these two quantified social costs (abbreviated as the SCM/SCC ratio) may be used as a metric to compare the relative importance of methane (or any other greenhouse gas) to carbon dioxide in creating the economic costs that result from climate change.
The social cost of any greenhouse gas is measured as the marginal damage of one unit emission of that particular gas, integrated over time. Here, the marginal damage is the additional amount of damage in economic terms (discounted over time) that is caused by one additional unit of emission.
Economists have tried to estimate the social cost of carbon for more than three decades. This research was pioneered in 1992 by William Nordhaus and William Cline in their respective work. Despite this long period of research, estimates of the social cost of carbon still span a wide range, quantified in rough terms from $10 to $1,000 per ton of carbon dioxide. The most important parameters that produce this wide range are the discount rate, damage function, and future temperature pathway. The US Environmental Protection Agency—building on work published in 2022 by the Social Cost of Carbon Initiative, a collaboration between Resources for the Future and the University of California, Berkeley—estimates a value of $190, following specific guidelines that were suggested in a 2017 report from the National Academies of Science, Engineering, and Medicine.
Efforts to estimate the social cost of methane have intensified lately (as with work from the Social Cost of Carbon Initiative in 2022 and our own work in 2023). In part, these efforts have been motivated by the increased recognition that multiplying the social cost of carbon by the GWP value for methane does not necessarily give an appropriate estimate of the social cost of methane.
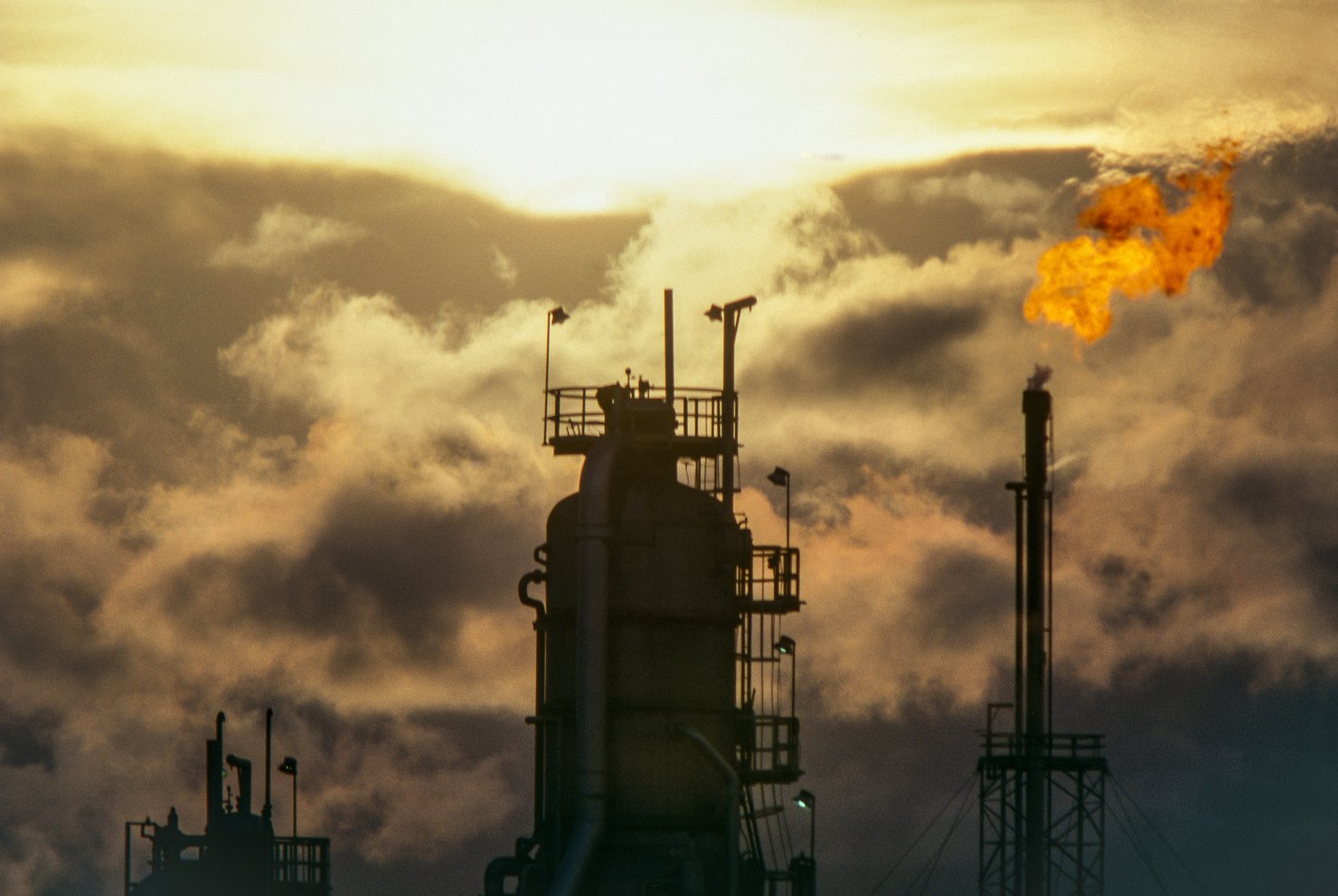
In our recent work, we estimate the social cost of methane and social cost of carbon. Our estimate of the social cost of methane is $4,000 per ton, with the social cost of carbon estimated as $192 per ton of carbon dioxide. The ratio of these two costs is 21, a somewhat lower value than the GWP100 of 27.
We find a higher estimate than Nordhaus for the social cost of carbon. The reason is that we use both a lower discount rate and a higher damage function. The lower discount rate is taken from a survey of economists and philosophers. A high discount rate implies that the lives of people in the future are worth less, just because they live in the future. The higher damage function comes from a recent meta-analysis of the costs of climate change. Our estimate of the social cost of carbon is roughly the same as that obtained by the Social Cost of Carbon Initiative, although our estimate of the social cost of methane is about twice as high.
Second, the SCM/SCC ratio varies with the discount rate. The lower the discount rate, the lower the ratio. The ratio drops because a lower discount rate increases the social cost of carbon much more than it increases the social cost of methane, given that carbon dioxide is much more long-lived in the atmosphere (Figure 1). When we use the higher discounting parameters from Nordhaus in our analysis (which entails a significantly higher discount rate), the SCM/SCC ratio increases to 33. This use of higher discount rates, which favors the present over the more distant future, is analogous to shorter time horizons in the GWP calculation.
A higher ratio implies that methane contributes relatively more to the costs of climate change, but a higher discount rate also means a drop in the social costs of both carbon and methane.
Our paper demonstrates a relationship between the SCM/SCC ratio and the time horizon of the GWP. Assuming that the background temperature pathway continues at close to current levels, we find that the difference between the discount rate and economic growth rate is inversely proportional to the time horizon. Thus, a discount rate of 1 percent above the growth rate yields an SCM/SCC ratio approximately equal to GWP100.
We also find that the social cost of carbon is sensitive to the background temperature. When we run our model with a business-as-usual emissions scenario that leads to a 3.7°C temperature increase by the year 2100, our estimate of the social cost of carbon increases to $1,200 per ton of carbon dioxide. This increase in the social cost of carbon stems entirely from the higher marginal damages that occur when the background temperature is higher.
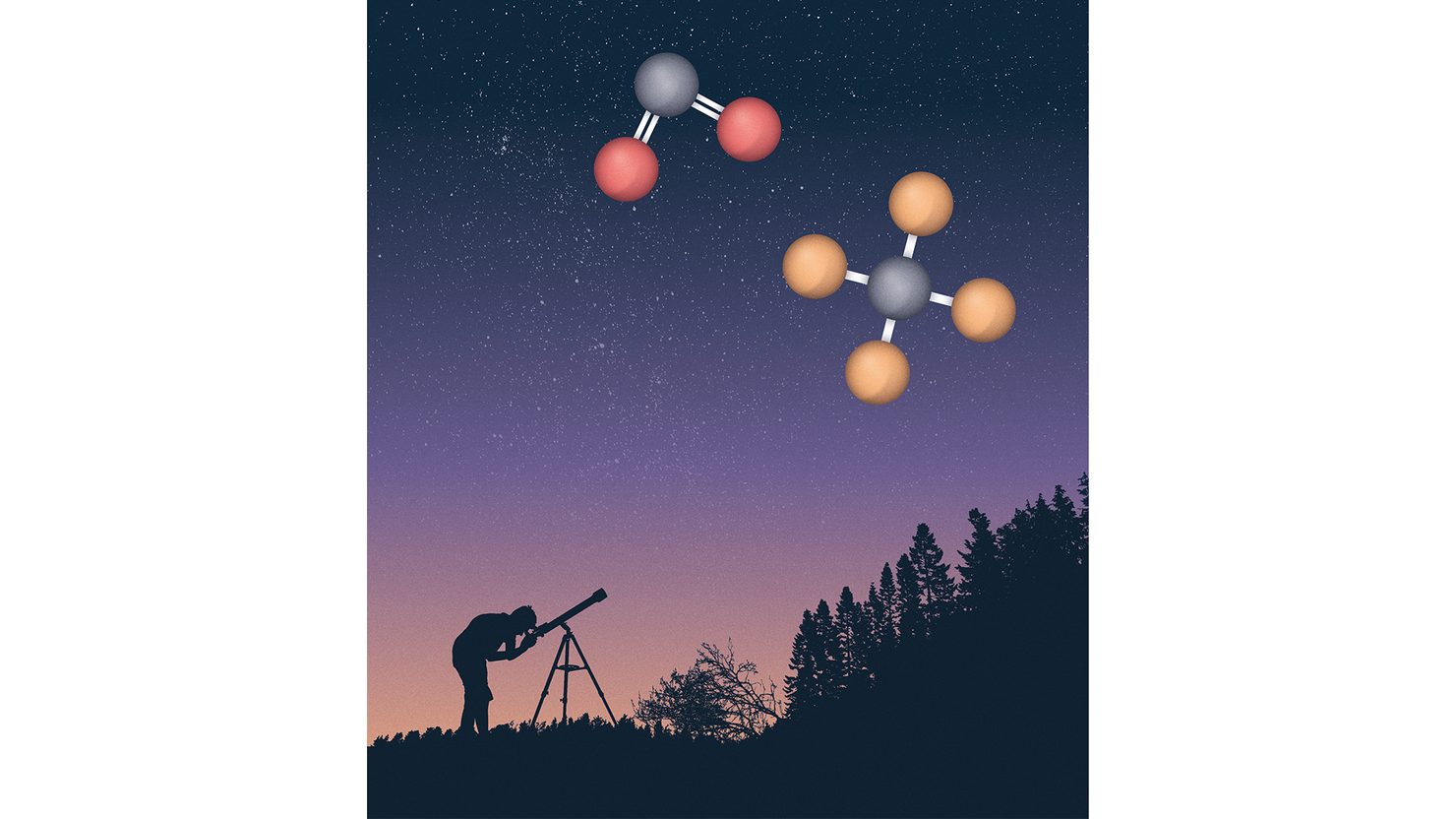
The social cost of methane also increases, by about a factor of two. This increase for methane is less than the increase in the estimate of the social cost of carbon because methane is short-lived and hence less affected by a long-term temperature pathway. The combined changes in the social costs of methane and carbon, in turn, means that the SCM/SCC ratio drops from 21 to around 7. Hence, with a higher background temperature, the social cost of carbon increases by several times, the social cost of methane doubles, and the effect of methane on climate change is significantly lower relative to carbon dioxide.
An interesting corollary here is that estimates for the social cost of carbon (in an optimizing framework, in contrast to predetermined temperature pathways) also depend on assumptions about the availability of negative emissions technologies (e.g., bioenergy with carbon capture and storage). When such technologies are available and cost-effective, the optimal temperature pathway may become significantly lower in the very far future, which would exert downward pressure on the social cost of carbon.
For instance, if we exclude the option of net-negative carbon dioxide emissions in our model, the estimate of the social cost of carbon increases from $192 to $318 per ton of carbon dioxide. The impact on the social cost of methane is much smaller (less than 10 percent), and the SCM/SCC ratio drops from 21 to 13.
Notably, for optimization models, the choice of whether to consider net-negative emissions in the long term significantly affects the optimal valuation of methane emissions vs carbon dioxide emissions.
Conclusions
Emissions of carbon dioxide from the combustion of fossil fuels are the dominant driver of climate change, while emissions of methane (whether from agriculture or fossil fuels) also play an important role. To meet the targets of the Paris Agreement, climate policies need to regulate both carbon dioxide and methane emissions. A key question is how much to value the mitigation of carbon dioxide emissions vs reductions in methane emissions.
Comparisons between methane and carbon dioxide are tricky and context dependent, with several pitfalls. Those who say that methane emissions do not have to be abated rely on the fact that methane emissions indeed can be sustained at a constant level even while maintaining a stable global temperature. However, every ton of methane emitted does increase the global temperature above what would otherwise be the case.
On the other hand, some say that methane should be given higher priority in efforts to mitigate climate change (in line with GWP20) because of the urgent nature of the climate problem. This argument risks downplaying the fact that climate change is quintessentially a long-run problem, a feature which is critical to take into account when considering carbon dioxide.
In this article, we have discussed two approaches to valuing the climate benefits of reducing methane vs carbon dioxide: calculating the GWP of these gases (an approach based on physics), and calculating a ratio of the social costs of the two greenhouse gases (a combined method based on physics and economics). Although different in many aspects, these two metrics are conceptually related. From an economics viewpoint, the most natural expression of the importance of each respective greenhouse gas probably is given by the SCM/SCC ratio, which leverages information about the relative impacts of greenhouse gases on society and welfare, not just the relative impacts on global temperature. On the other hand, policymakers should note potential complications associated with the uncertainty of variables such as discount rates and temperature trajectories.