Resources Radio, a podcast produced by the Resources editorial team and Resources for the Future (RFF), broadcast its 200th episode last fall. New episodes are released weekly, in which one of the hosts—Daniel Raimi, Kristin Hayes, or Margaret Walls—speaks with a special guest about energy, environmental policy, climate impacts, and more.
Transcribed here is one such episode, in which Alex Gilbert catches us up on recent advances in nuclear technologies and how recent policies—especially the Inflation Reduction Act—are incentivizing nuclear energy deployment. Gilbert leads regulatory efforts at Zeno Power for applications of nuclear energy systems in space. He’s a fellow and PhD student at the Payne Institute for Public Policy at the Colorado School of Mines.
Listen to the Podcast
This interview was originally released on September 27, 2022. The transcript of the conversation has been edited for length and clarity.

Daniel Raimi: Can you tell us how you started working on energy and environmental topics in the first place?
Alex Gilbert: If you care about environmental issues and climate change, then what you really care about is energy. It’s your top concern, because energy has the biggest impact on the environment out of anything that humans do. Conversely, if you care about energy systems, you care about the environment, because the environment is the largest constraint on energy systems. I started focusing on energy systems because of the environmental aspect.
Nuclear energy in the United States and globally is a very large energy source. In the United States, it’s the single largest clean power source. Even today, hydro, wind, and solar power combined produce about as much energy as just nuclear. Globally, about 10 percent of all power is from nuclear energy. Next to hydropower, nuclear is the second-largest clean energy source globally.
Your role at Zeno Power involves nuclear energy and related regulatory work—so, you are participating in the nuclear economy. Do you want to say anything about disclosures regarding your work?
Zeno Power is developing radioisotope power sources. We essentially take nuclear waste and use it to create small power sources for outer space and for remote locations on Earth. Historically, this is how people have powered things like the Mars rovers and deep-space probes.
We at Zeno see ourselves as part of this waste-innovation group that is emerging right now. Various companies are starting to say, “We’ve seen all this innovation happening with nuclear reactors, and it’s important for emissions reductions and for the success of the nuclear industry, but we’re still going to have a challenge with nuclear waste. What can we do with that waste? What are ways to recycle it or to otherwise address it—to potentially store it, from a commercial perspective, that’s longer term?”
As for the nuclear industry more broadly, we definitely are rooting for its success, but our company is separate from the innovations that are happening right now with advanced nuclear reactors.
We’re going to talk about this bucket of technologies that you’ve referred to as “advanced nuclear technologies” and what the private and public sectors have been doing to push those technologies forward in recent years. Can you define “advanced nuclear technologies” for us?
It’s a very squishy term. If you talk to different nuclear energy professionals, you’ll hear different definitions. The best way to think about advanced nuclear technology is it’s everything that’s not a large light-water reactor, which is what exists right now. The United States is building two AP1000 nuclear plants in the South. Those are the last of the non-advanced reactors. Everything else that we’ll build moving forward is considered, more or less, an advanced reactor.
What does that mean from a technology perspective? To start with basics, nuclear fission is based on the fission of atoms to create energy. You take a fissile isotope (an atom), usually uranium-235, and you hit it with a neutron. That neutron causes the atom to split. That split creates what we call “fission products,” which are one of the main things in nuclear waste that we’re concerned about. But it also creates energy, which we can harness. And it creates more neutrons, usually two or three, that you can then use to create more fissions.
A fission chain reaction can produce energy sustainably. And because we’re dealing with atomic bonds here, and not chemical bonds, there’s a lot more energy released than, say, burning natural gas or burning coal.
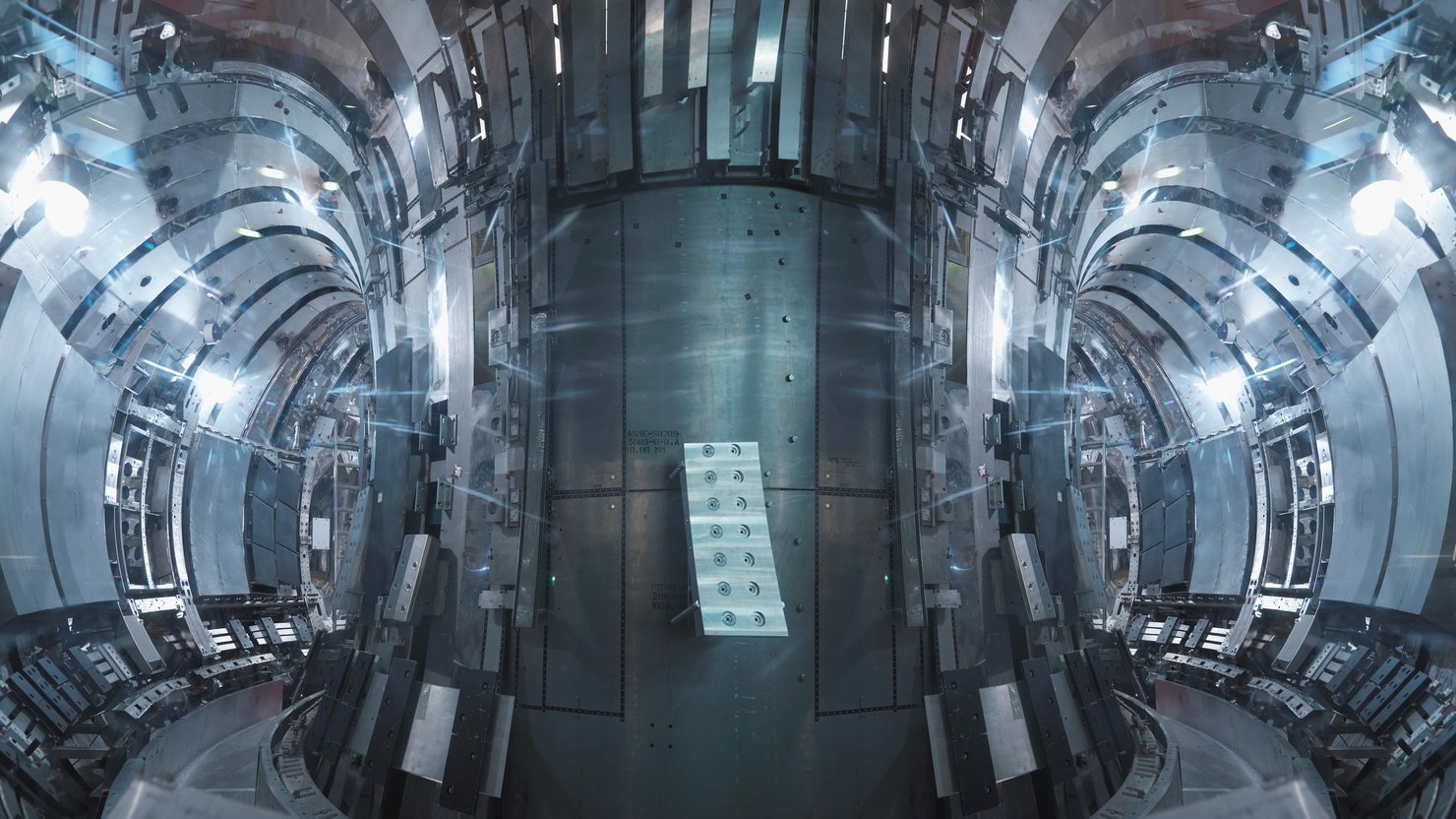
Historically, the way that we’ve harnessed that large load of energy is with a large light-water reactor. What does that mean? Generally, these are reactors that are a gigawatt or more in capacity. They use the isotope uranium-235 in fuel rods. Uranium-235 actually produces the fission, but this isotope accounts for only about 5 percent of the fuel rods; about 95 percent of the uranium in fuel rods is uranium-238, which doesn’t fission much. That enrichment with uranium-235 is a key part of how you run these reactors.
The design philosophy with nuclear is about efficiencies of scale. The larger the reactor is, the more you can get the costs down in terms of engineering, regulatory costs, equipment, and materials.
When you run a nuclear reactor, you’re using water for several things. Water transfers heat in either pressurized- or boiling-water reactors. It’s like a coal or natural gas plant—you’re using steam to turn a turbine. But you’re also using the water to cool the fuel itself. The fuel heats up over time; if you don’t cool it, it will cause issues like meltdowns and other sorts of accidents.
The other important thing with water is that it’s a moderator: it slows down neutron speeds. Neutrons have different speeds. If they hit an atom at a certain speed, they’ll cause the atom to fission. If they’re going too fast or too slow, they might not cause fission.
What does all this mean in terms of advanced nuclear reactors? Advanced reactors essentially are everything that’s not what I’ve just described to define large light-water reactors.
One class of advanced reactors is called “small modular reactors.” This class is similar to large light-water reactors in that small modular reactors use fuel rods with the same uranium enrichment levels. They use water as the coolant, heat-transfer mechanism, and moderator. But these reactors are smaller: instead of one gigawatt, we’re talking anywhere from 50 megawatts to a couple hundred megawatts. With small modular reactors, you’re trying to get efficiencies of serial production instead of trying to get efficiencies of scale.
Aside from the small modular reactors, other types of advanced reactors are quite different. They use different fuel forms and different chemical setups for the fuel. They don’t use fuel rods. Some of them have different enrichment levels. Instead of 5 percent uranium-235, they go up to 20 percent uranium-235. Some reactors have different coolants. They don’t necessarily use water. They might not need moderators, because instead of needing to slow down the neutron speed, they use what’s called the “fast spectrum.” Their design uses quicker neutrons that fission more efficiently.
Others include molten-salt reactors. High-temperature gas reactors use a type of fuel that’s essentially little billiard balls of uranium instead of fuel rods. We also have liquid-metal cooled reactors. These various advanced reactors can fission in different, more efficient, or more economical ways.
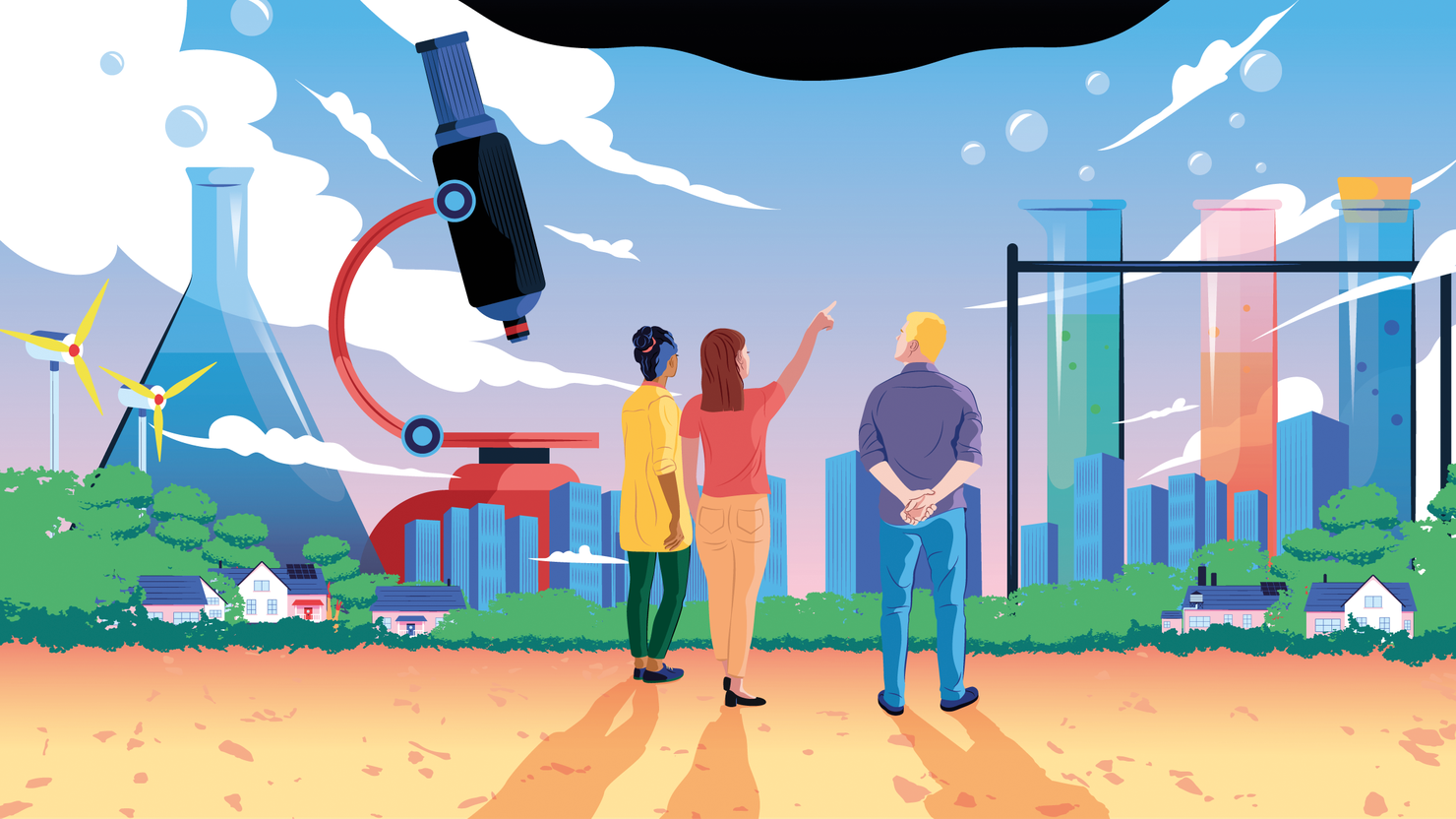
We also have a new category of reactors called “microreactors.” These are defined by their size, instead of according to the way they fission atoms, their fuel form, or their enrichment levels. Traditional large light-water reactors are one gigawatt. Small modular reactors are maybe 50 megawatts to 200 megawatts or more per reactor. Other advanced reactors are somewhere on the scale of hundreds of megawatts. Microreactors are much smaller. Commercial reactors can be as small as 1 megawatt, with many in the 5–10 megawatt range, and some up to 20 or 30 megawatts. This is completely different for the nuclear industry. It’s distributed nuclear energy on a distributed scale. You could use this type of energy for small towns, on the grid’s edge, or for remote operations.
These new advanced reactors are opening up new ways of using nuclear energy.
Can you help us understand some of the differences, with regard to economics, safety of operations, and waste when we think about the large light-water reactors of today versus these next-generation technologies that are being discussed and developed?
Economics is probably the most important difference. That’s because, once large light-water reactors are built, they’re very cheap to operate, and you can operate them for decades. The problem is building them in the first place. They are megaprojects. They cost billions of dollars. They can take a decade or more to build. That is a challenge to finance, especially with what we’re seeing with electricity markets: increased competition and moving away from rate-basing.
On the economics side, the advanced-reactor companies are trying various methods. First of all, many advanced-reactor companies exist. It’s a very different situation to have competition emerging among developers. Instead of trying to get efficiencies of scale by making big reactors, these new companies are trying to go for the economics of series production—essentially, looking at how wind and solar were able to iterate rapidly over multiple generations to drive costs down.
In the United States, we’ve never had “nth” of a kind of reactor built. Everything that we’ve done is essentially a first-of-a-kind, bespoke reactor. That leads to significant increases in costs, as well as construction complexity and issues arising.
The biggest motivator for the innovation among these new reactor companies is to get economies of scale and reduce nonrecurring engineering costs, so that it’s much cheaper and more affordable to build these reactors than ever before.
On the safety side, we’re also seeing major advancements. Advanced reactors all use a different principle to get to what’s called “inherent safety.” The idea is that you don’t need active systems to function to keep the reactors safe. Those active systems are things that we’ve seen to be problematic in all major accidents. At Fukushima, in particular, the reactor lost off-site power supply. Their basement generators flooded, which prevented them from running water over the reactors to cool them, which eventually led to the accident.
Advanced reactors completely change all elements of the risk equation, which for industrial risk is the consequence of an accident times the probability. The consequences are going to be a lot smaller for advanced reactors because of their design. One, they’re smaller; there’s less nuclear material at risk. Two, they don’t necessarily run at higher pressures and don’t need to have consistent cooling. They don’t need as many operator interventions. Three, they reduce the likelihood of any accidents happening in the first place by being simpler systems and by requiring less intervention.
No advanced reactor built in the United States will be less safe than the existing fleet, because of regulation. Safety regulation will use standards for conventional light-water reactors as the baseline benchmark. If you run the numbers, next-generation reactors will be at least one to two or more orders of magnitude safer than that.
One of the issues with nuclear is that it’s on a longer time frame.
Finally, waste. Waste is more of an uncertainty. It’s an area that’s not driving innovation as much as economics. As we’ve scaled down the reactors, we’ve been losing some of the efficiencies of scale for waste production. We can expect to see more low-level or intermediate waste, which we can handle with the existing waste system—but there’s going to be more of it.
The big concern is high-level waste—spent nuclear fuel. We could see some increases in the volume of high-level waste—or it’s possible that the volume of waste will be about the same as with the existing large light-water reactors. That’s uncertain. It also depends on how the fuel cycle works and seeing if we can find any innovations on that front. Ultimately, though, we do manage nuclear waste responsibly in the commercial sector. We have short-term solutions for the waste problem, but we will need a geological repository for any type of nuclear energy, including advanced reactors. This is still a major policy challenge.
Let’s move to what’s happening in the real world. Various demonstration projects are in the works for nuclear technologies that are in development. Can you give a few examples of what these projects may look like or even plants that are operational?
Not quite two years ago, when I was at the Nuclear Innovation Alliance, I worked with some other nongovernmental advanced nuclear organizations—Third Way, Clean Air Task Force, and ClearPath—to ask, “Beyond just ideas, are these projects the real thing? Are they moving forward?” We found that just over 30 advanced reactors around the world are in what we would consider advanced stages of demonstration, several of which are operating.
To start off, the United States built and operated a very small advanced reactor in 2018 called Kilopower. It was a kilowatt-scale reactor that demonstrated the capability of something like this for NASA and space applications. That was important, because it was the first new reactor that was designed, built, and operated in the United States in decades. That project kind of kicked off things here. Its design philosophy has inspired a lot of the companies that are developing reactors in the United States.
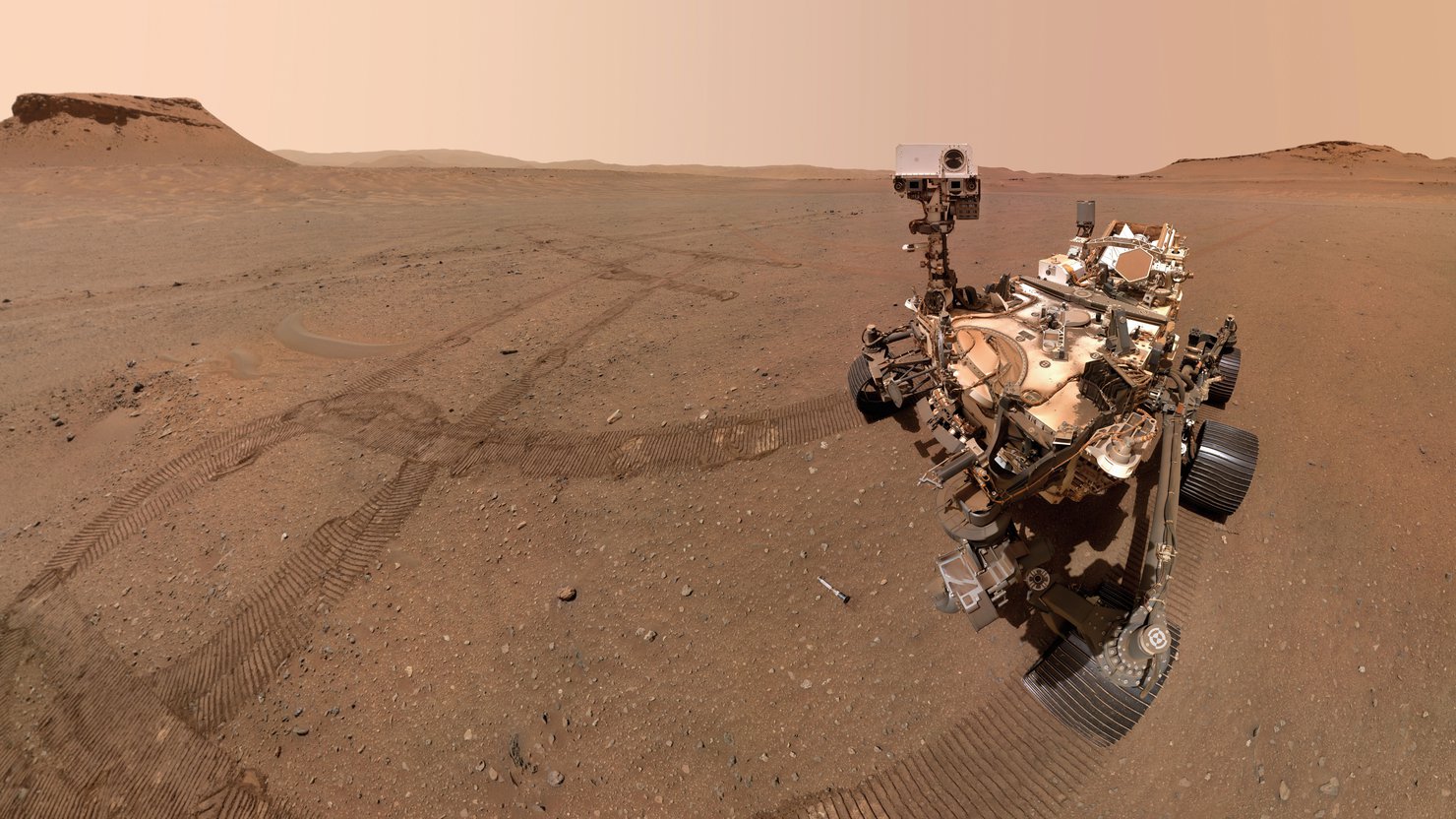
Abroad, projects are in operation. Russia has a small modular reactor, a light-water reactor called the Akademik Lomonosov, which is derived from some of their nuclear technologies. It’s essentially a floating nuclear power plant—a barge that’s currently powering a small town in the Russian Arctic. In addition to providing power, it’s also providing heat. That’s really important if you’re trying to look at the decarbonization potential. Earlier this year, China officially opened their high-temperature gas reactor that they’ve been working on for several decades. They’ve got that demonstration project running, along with other projects that are in advanced licensing and construction phases.
In the United States, we’re right at the point where we’re starting to license reactor projects commercially. We have three major demonstration projects: TerraPower and X-energy have funding from the Department of Energy’s Advanced Reactor Demonstration Program. They’re going to submit their first license applications within a year or so—one, to build a reactor in Wyoming at a retiring coal plant; two, to build a reactor for industrial heat on the Gulf Coast. NuScale Power, which recently went public, is working to build a reactor to power Utah utilities, as well. These three projects are set to be operating by 2030.
We also have a number of small-scale projects—Kairos Power, Oklo, and Ultra Safe Nuclear Corporation—that are moving toward developing different types of projects. Some of them will focus more on the research scale. The Kairos Power project, which is undergoing licensing right now, is meant to provide information for a larger version that they’ll do down the line, whereas the Ultra Safe project is a research reactor to support research activities at the University of Illinois.
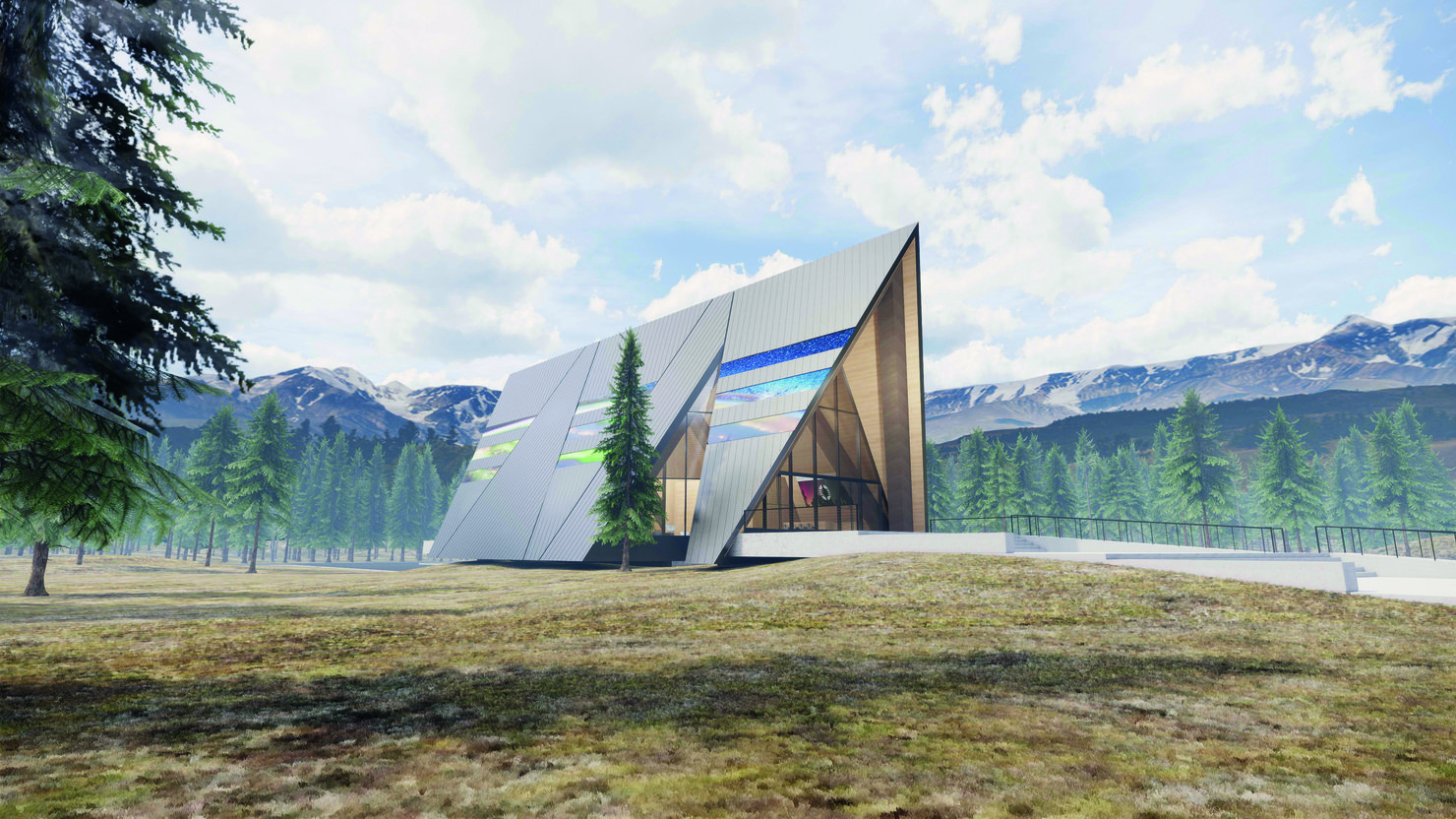
One other big demonstration project that I want to flag is Holtec, a longtime nuclear company. They’ve just announced applications for over $7 billion in loan guarantees to build a factory for the first four of its reactors and ultimately to produce more reactors.
We’re seeing a lot of momentum to get to the first major stage, which is licensing. We would expect that a lot of these initial small reactors will be online by the middle of the decade, with the larger reactors online by the end of the decade.
What about policy here in the United States? A lot of legislation has passed in the last five years or so that has facilitated nuclear energy in different ways. Can you highlight a few ways in which these new pieces of legislation seek to accelerate the deployment of different kinds of advanced nuclear technologies?
It really started off in 2017 or 2018 with work on two precursor bills: the Nuclear Energy Innovation and Modernization Act, which focused on making the Nuclear Regulatory Commission into a modern regulator, and the Nuclear Energy Innovation Capabilities Act, which focused on bringing the Department of Energy up to speed for advanced reactors. These pieces of legislation kick-started innovation at the Nuclear Regulatory Commission and the US Department of Energy.
The idea with the Nuclear Regulatory Commission was that we’ll need to reform how we regulate nuclear energy. The way our entire regulatory system is set up is around large light-water reactors. To handle the whole variety of advanced reactors, we need to reform how we make smart, effective regulations so we can reassure the public that we are handling these reactors safely.
The Nuclear Energy Innovation Capabilities Act is the beginning of a lot of related work at the Department of Energy; this legislation ensures that the agency has the capability to support innovation. Since then, almost every major energy bill that we’ve seen in the last several years has had some nuclear component: the Energy Act of 2020, the Infrastructure Investment and Jobs Act, the Creating Helpful Incentives to Produce Semiconductors Act, and the Inflation Reduction Act. The Inflation Reduction Act has been the keystone that capped off this whole area of very active legislation. Most of these bills have been bipartisan; both parties are supporting nuclear right now. Both parties see nuclear as an area that everyone can work on together because of its clean energy and decarbonization potential.
An important point is that these programs have established the basis for demonstration projects, either through direct funding or, most recently with the Inflation Reduction Act, by setting up the tax credits that will allow us to build many of these reactors in the future. The Inflation Reduction Act levels the playing field for advanced nuclear reactors and other clean energy sources.
You highlight the provisions of the Inflation Reduction Act as particularly important. How is the Inflation Reduction Act likely to benefit not just next-generation reactors, but also the fleet of existing reactors, many of which have retired in recent years or have been in other types of economic straits?
The primary way the Inflation Reduction Act benefits nuclear energy is via tax credits. This is the case for advanced nuclear energy, specifically: the act establishes tax credits that essentially are equal to the credits that exist for other clean energy sources out there.
These tax credits are valuable because advanced nuclear projects are still in the first-of-a-kind development stage. They’ll need support from tax credits to get to market, compete, and scale up until they become more self-sufficient. Other small tax credits throughout the Inflation Reduction Act could help: the Advanced Manufacturing Production Credit; some of the support for hydrogen tax credits; and provisions that are more focused on demand or on other opportunities, which could include nuclear.
In the United States, we’re right at the point where we’re starting to license reactor projects commercially.
Another big way that the Inflation Reduction Act benefits nuclear is by providing support for the existing nuclear fleet. Over the past 10 years, we’ve seen a large number of retirements in the existing nuclear fleet—we’ve had over 10 gigawatts retire. The nuclear fleet is the largest single source of carbon-free power in the United States, which has been a big concern for state and federal policy for years. We’ve seen a lot of work to try and reverse those retirements. The Inflation Reduction Act, working in conjunction with some provisions in the Infrastructure Investment and Jobs Act, provides funding that targets the nuclear reactors that are most at risk of retirement.
Within several weeks of the Inflation Reduction Act passing, the Diablo Canyon nuclear power plant in California, which was set to close down, completely flipped from having political opposition for any sort of long-term operations, to having significant political support, including from the governor, for long-term operations. The funding from both the Inflation Reduction Act and the Infrastructure Investment and Jobs Act has seemed to change the direction of that facility.
We’ve also seen that the Palisades Nuclear Generating Station in Michigan, which closed down in May 2022, is looking at restarting and coming back online. Many people, even the nuclear industry, did not expect that to happen. The funding and the Inflation Reduction Act already are having impacts on markets.
How about expectations for the future: If you had to speculate about the future of nuclear energy in the United States, where do you think it’ll be in 5 or 10 years?
For a long time, people thought that nuclear production in the United States would decline steadily through the middle of the century as reactors continued to retire. With recent policy developments, it seems right now that nuclear power from existing power plants probably will remain largely flat. We don’t expect markets to drive many more retirements in the next 10 years or so.
The big question is, What happens on the advanced nuclear side? One of the issues with nuclear is that it’s on a longer time frame. It takes a while to build these projects and get them established, but they’ll operate for a very long time once they’re built.
Over the next 10 years, I think a couple things will happen. First, we’ll see microreactors—the 1- to 30-megawatt reactors—grow and accelerate quickly. The project life cycles on those are really short. In theory, right now, the primary determining factor for the timeline is licensing. Once you have production capabilities, you probably can get a microreactor up and going within 12 months in terms of construction. We could see a large number of microreactors being built in locations throughout the United States, particularly in Alaska.
In terms of the broader energy markets, we’re likely to see a large amount of orders for advanced reactors. Especially as these demonstration projects move closer to operation by the end of the decade, I expect that we’ll start seeing advanced nuclear considered more in integrated resource plans and utility-level analysis.
When you’re looking at the decarbonization potential—and how much this type of energy generation can grow—we won’t see a lot in the 2020s. We’ll see that more in the 2030s, with rapid acceleration in the 2040s if the industry takes off; that is, if the nuclear industry can solve its economic challenges.
That’s one of my big takeaways from the Inflation Reduction Act. The policy environment now is largely complete. The federal government, working with its regulatory and other entities and with state governments, largely is setting things up for industry to deliver. It’s going to be on industry to show that they can build these projects on time, on budget, and economically.
Various recent studies have shown that the potential, if the industry can deliver, is literally hundreds of gigawatts. By the middle of the century, we could see advanced nuclear reactors dwarfing the scale of the existing nuclear fleet—we could see 100 to 400 gigawatts. This progress could decarbonize not just power, but also industrial heat and process heat.
That’s the potential—but we’re not going to know whether industry has cracked that nut for quite a while. It’ll be a big challenge for them to get to that scale, especially in these time frames.