This is the second part of a two-part article by the director of RFF' s new Climate Resources Program. The article—the first part of which appeared in Resources, Winter 1987—is drawn from the author's RFF discussion paper RR86-04, "A Primer on Climate Change: Mechanisms, Trends and Projections," the full and annotated text of which is available prepaid at $3.00 (including postage) from the Renewable Resources Division, Resources for the Future, 1616 P Street, NW., Washington, D.C. 20036.

Atmospheric turbidity—any condition of the atmosphere that reduces its transparency to radiation, especially visible radiation—affects our climate. Ordinarily the term turbidity is applied to the cloud-free portions of the atmosphere. Pollens, dusts, smoke, water vapor, and all suspended materials affect the atmosphere's turbidity. The term aerosol is used to describe dispersed solid or liquid particles suspended in the air.
Volcanism is the greatest natural contributor to the changing turbidity of the atmosphere, although other natural phenomena such as dust storms and forest and range fires also make significant contributions at times. Slash-and-burn agriculture and other land-clearing and residue-burning operations also load considerable amounts of aerosol into the atmosphere. Plants, too, exude organic aerosols; blue haze over forest areas has been attributed to such emanations.
Through his management or mismanagement of soils and vegetation, man affects the magnitude of atmospheric loading from all these sources except volcanism. In addition, power generation, industrial combustion processes and space heating, and refuse burning load large quantities of soot and other materials into the atmosphere.
Much has been written in recent years about the possibility that increased atmospheric turbidity may be changing climate. Some scientists have related volcanic eruptions to specific climatic events. Experts have also suggested that increasing atmospheric turbidity might have been the cause of the Sahelian droughts in the 1970s.
Evidence now suggests, however, that the earth's atmosphere is not becoming more turbid. As measured at the observatory on Mauna Loa mountain in Hawaii, there have been no long-term changes in the amount of small particles suspended in the air sampled over the island. Located in the mid-Pacific at an altitude of 3,350 meters (about 11,000 feet), the air sampled at the observatory represents the condition of the mixed or "average" global atmosphere exceptionally well.
The transmissivity of the atmosphere has been affected from time to time, however, by explosive volcanic events that eject debris into the troposphere and sometimes into the stratosphere. (The troposphere is the lowest layer of the atmosphere, extending on the average to about 15 kilometers above the earth; the stratosphere is the layer of the atmosphere extending upward from the top of the troposphere to between 20 and 25 kilometers.) When the stratosphere is relatively free from volcanic intrusions, the transmissivity of the upper atmosphere remains within a relatively limited range. It must also be borne in mind, however, that the turbidity of the atmosphere is frequently increased by aerosols generated by dust storms, the burning of vegetation, and industrial combustion processes. These materials, which tend to settle out of the atmosphere after a few weeks, usually affect radiation balance on a local or regional scale.
The recent episodes of volcanic activity at Mount St. Helens in Washington State and El Chichon in Mexico had quite different effects on the atmosphere. The eruption of the former in 1980 injected immense quantities of relatively large silicate and pumice particles mostly into the troposphere. But some materials were injected into the stratosphere as well. While they remained suspended, these materials certainly could have increased atmospheric reflectivity to space, and they did result in a measurable but transitory reduction of solar radiation receipts at the ground.
By contrast, the El Chichon eruption in 1982 injected great quantities of sulfurous gases into the stratosphere. These were converted to sulfuric acid particles, which can remain suspended for a number of years. Reductions in solar radiation received at ground level were measurable at a number of sites as the volcanic cloud spread over the globe. Residual effects of El Chichon are still measurable.
Trace gases and the "greenhouse effect"
The earth's surfaces, in order to dissipate the energy received from the sun, reradiate in the longwave, or infrared, band from about 3.0 to 80 micrometers. Most of the emission occurs in the wave band from 8 to 14 micrometers, with the wavelength of maximum emission at about 10 micrometers. Water vapor, a strong absorber of infrared radiation, is somewhat transparent in the 8-to-14-micrometer range. This wave band is called the atmospheric window.
Methane, nitrous oxide, ozone, carbon dioxide, the chlorofluorocarbons, carbon tetrachloride, and carbon disulfide all have absorption peaks that tend to close the atmospheric window. At this time, probably as a result of man's activities, the concentration of each of these infrared absorptive gases is increasing in the atmosphere. Methane, a gas produced under anaerobic conditions, has been increasing at the rate of about 1 percent per year for the last decade or so. Current concentration of this gas is about 1.65 parts per million by volume. Ice-core data indicate that the atmospheric concentration of methane may have increased steadily over the last several hundred years. One likely cause is the growth of rice paddy culture around the world. As methane is also a product of both ruminant and nonruminant digestive systems, increasing numbers of animals may account for part of the increase. It has been shown, too, that methane is an important end-product of cellulose digestion by termites. The rapid increase in methane is also a result of the accumulation in the atmosphere of other gaseous species such as carbon monoxide which react with hydroxyl (OH) radicals that would otherwise reduce the methane molecules.
A great increase in the use of nitrogen fertilizers in agriculture, especially since World War II, has been implicated in the average annual 0.2 percent increases of nitrous oxide observed in the atmosphere. Nitrous oxide is released from soil to the atmosphere during the nitrification of ammonium-producing fertilizers under aerobic conditions. However, nitrous oxide is also emitted as the result of denitrification—a process that occurs when soils become waterlogged. It has been suggested that the drainage of such soils and alterations in their acidity may have reduced this source of natural nitrogenous emissions to some degree. The combustion of nitrogen-rich fossil fuels may also be contributing to the increase in nitrous oxide.
Until now the trace gas that has received most scientific and public attention is carbon dioxide, an end-product of fossil fuel combustion, cement manufacture, and respiration by living organisms. It's concentration in the atmosphere has increased since at least the beginning of the industrial revolution. Since the late nineteenth century, scientists have speculated that because of its strong absorption of infrared radiation, especially in the atmospheric window, the rising concentration of carbon dioxide must cause a warming of the lower layers of the atmosphere. This phenomenon has been likened to the process that occurs in greenhouses: the glass permits solar radiation to penetrate, but it absorbs infrared radiation emitted by the soil and plants within. Although the analogy is defective, the process of warming in the lower layers of the atmosphere caused by the infrared absorption behavior of carbon dioxide and the other radiatively active trace gases mentioned above has come to be known as the "greenhouse effect." It is not known for certain what the concentration of carbon dioxide was before the industrial revolution, although the record of sporadic air analyses from 1870 on and samples of air trapped in glacial ice suggest a value of around 280 parts per million.
In 1958, as one of the activities undertaken during the International Geophysical Year, continuous observations of atmospheric carbon dioxide concentration were begun by the Scripps Institute of Oceanography on Mauna Loa (see figure 2). The observations reveal a continuous and possibly accelerating secular increase in the mean annual concentration: in 1958 the concentration was about 316 parts per million; at the end of 1985 it was 345 parts per million. The annual cycle shown in the figure is a response to the seasonal photosynthetic activity in the Northern Hemisphere: during the growing season more carbon dioxide is drawn out of the atmosphere by photosynthesizing plants than is released into it by respiration; in winter the opposite is true. The records of observations of carbon dioxide concentrations at the South Pole, also started in 1958, are less continuous than those from Hawaii, but they closely confirm the findings at Mauna Loa, as do the measurements made at a number of other, more recently established stations around the world. All of these records suggest that the increase in atmospheric carbon dioxide concentration is a global phenomenon. They indicate that in the course of only a quarter-century, carbon dioxide concentration in the atmosphere has increased by nearly 10 percent. And if we consider plausible carbon dioxide concentrations before the industrial revolution, there has been at least a 23 percent increase in carbon dioxide in the atmosphere since then.
Whether the causes of this increase are fossil fuel combustion and cement manufacture alone is not certain. More likely, the great changes in land use affected by rain—the destruction of forests and their conversion to urban areas, grasslands, and farmlands, and the breaking of sod in natural prairies and plains to permit crop culture—have also been responsible for adding large amounts of carbon dioxide to the atmosphere—carbon dioxide being the end-product when trees are burned and the above-ground litter and soil organic matter are oxidized by microorganisms.
Figure 1. Global annual mean temperature variations since 1861, based on land and marine data
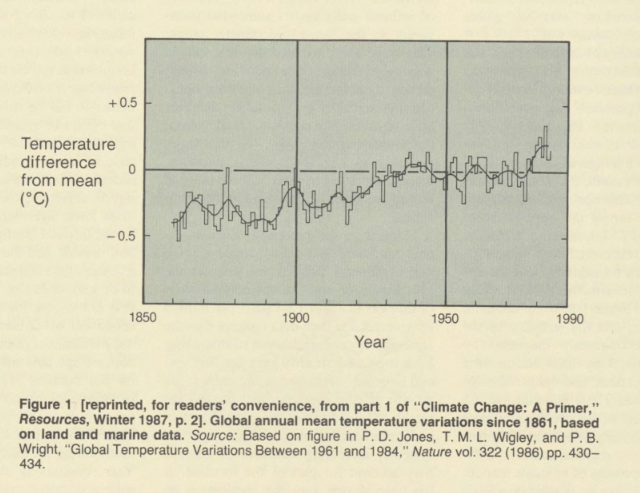
Figure 2. Concentration of atmospheric carbon dioxide at Mauna Loa Observatory, Hawaii
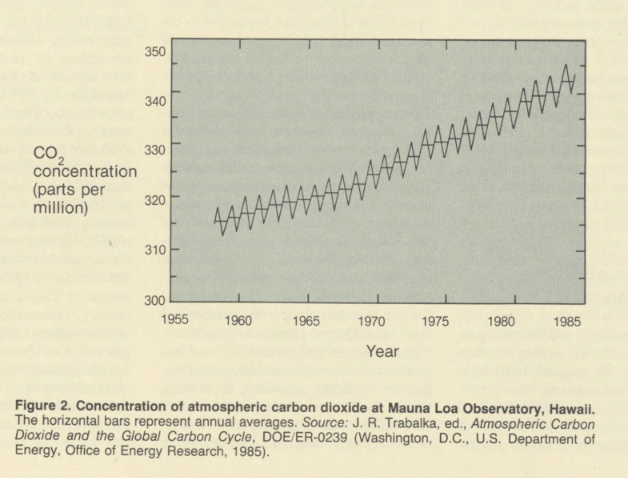
It is uncertain whether land use changes today continue to add significant amounts of carbon dioxide to the atmosphere. Concern has been voiced that conversion of tropical rainforests in Amazonia and elsewhere may lead to additional large increments in atmospheric carbon dioxide. However, the extent of current forest conversion rates is unclear, and the regrowth of forests in the temperate regions may offset these releases to some degree.
Industrial processes are probably the overwhelming cause of the carbon dioxide increase in the atmosphere at this time. Calculations of worldwide fossil fuel usage indicate that the equivalent of at least 3 parts per million of carbon dioxide are injected into the atmosphere yearly. Yet the Mauna Loa record shows that the annual increase over the last ten years has been about 1.36 parts per million. Thus, at least 1.64 parts per million—and, if non-fossil-fuel sources are significant, more—carbon dioxide is unaccounted for.
Some carbon dioxide is recaptured by oceans and on land. Although the matter is disputed, there is some evidence that, despite deforestation in the tropics, reforestation and regrowth of cutover areas may be increasing the area of forested land in the temperate regions. (This conclusion was reached by RFF senior fellows Roger A. Sedjo and Marion Clawson, and reported in their chapter "Global Forests" in The Resourceful Earth [Oxford, Blackwell, 1984].) If this is so, the new trees, to support their growth, may actually be extracting large amounts of the excess carbon dioxide from the atmosphere. Other vegetation may also be fixing more carbon dioxide, since photosynthesis increases proportionately in most species with increasing concentration of carbon dioxide in the ambient air.
The role of the biosphere in regulating carbon dioxide concentration in the atmosphere is not yet well understood. In fact, it is uncertain which of the world's major ecosystems are net sources of atmospheric carbon dioxide and which are net "sinks" for it. Whether the world's terrestrial ecosystems as a whole are a net source or a net sink for atmospheric carbon dioxide is also the subject of dispute.
Land use change
Humans have affected the radiation balance of large tracts of earth through changes in land use that lead to changes in the reflection of solar radiation. For example, new snow may reflect 90 percent or more; sandy desert may reflect 50 percent; dark dry soil, 15 percent; the same soil when wet, 8 percent; an alfalfa field, 24 percent; a coniferous forest, 20 percent; calm ocean, 7 percent; wavy ocean, 14 percent; a silt-laden river, 12 percent. Changes in land use also cause changes in surface temperature. The latter affects the emission of longwave radiation to the atmosphere and space.
Most often we think of land use change as due to man's activities. The condition of land surfaces may change as the result of natural processes as well. Soil erosion and species succession alter the character of the surface; these processes may be initiated by geological events, by evolutionary pressures, and, of course, by climatic change. The extent to which these conversions may alter local climate can be illustrated with a few examples. Consider the conversion to grassland of a hectare of land in the middle of a forest. Surface reflectivity for solar radiation will increase from, say, 18 to 24 percent; the effect will be a reduction in the net radiation. Trees that are deep-rooted can usually continue to extract water after shallow-rooted grass has exhausted its supply. Transpiration is the primary mechanism that allows leaves to remain cool even as they absorb the shortwave and longwave radiation that impinges on them. Thus, the mean temperature of the forest at noon might be 25° C compared to 35° C for grass under the same weather conditions. This difference in leaf temperature increases outgoing longwave radiation by about 14 percent, further reducing the net radiation over the grass.
Figure 3. Departures from normal rainfall in sub-Saharan Africa, 1941-1986
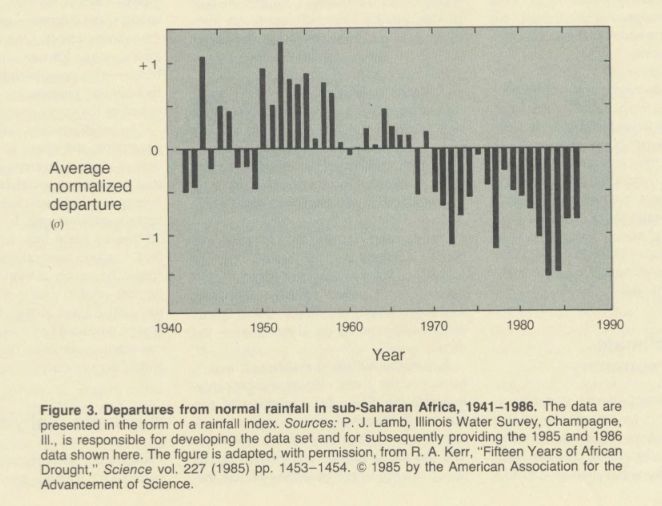
The net radiation or available energy is not necessarily partitioned in the same ways in grassland and forest. After the grass has exhausted the water from its shallow root zone, its transpiration rate is sharply reduced. The surface becomes warmer than the air above and transfers heat to it. As the air above the grass becomes warmer, its ability to hold water vapor, an exponential function of the air temperature, increases. Relative humidity falls. The warm dry air over the grass can be carried by the wind into the adjacent forest. There, if soil water is still available to the tree roots, it may supplement the energy from the sun and increase the rate of evapotranspiration above that prevailing in the rest of the forest.
The example given above describes the effects of land conversion at the micro-scale. The same principles apply when large areas of land undergo alterations in use. When rainfed agricultural lands are irrigated, when forests are removed, when rangelands are overgrazed and denuded, even when portions of large lakes or seas undergo color changes due to algal blooms, their radiation and energy balances are altered, with consequent effects on the local climate and on the climate of adjacent lands and waters downwind.
Factors in climate change—a summary
Three major causes of climate change have been described thus far: (1) changes in the supply of solar energy, (2) changes in the transmissivity of the atmosphere for both incoming and outgoing radiation, and (3) changes in land use that alter the radiation balance. Other causes include the release of heat (thermal pollution) that warms the lower atmosphere directly; the upward transport of chlorofluoromethanes and nitrous oxide into the stratosphere, where photochemical reaction of their dissociation products probably reduces stratospheric ozone with a consequent increase in ultraviolet irradiation of the surface and its inhabitants; and the release of trace gases such as nitrogen oxides, carbon monoxide, or methane that increase ozone concentration in the troposphere by photochemical reactions. Tropospheric ozone also causes significant atmospheric heating that enhances both solar and greenhouse heating of the lower atmosphere.
Change is an inherent feature of the earth's climate. In the future as in the past, solar luminosity will fluctuate in ways that are only partly predictable. Cycles in the orbital geometry of the earth-sun system will proceed inexorably. Volcanism will be the primary cause of significant fluctuations in the transparency of the atmosphere to solar radiation. Over these phenomena man has no control.
The earth is currently about 10,000 years into an interglacial cycle. There is no valid reason to assume that the final ice age has been experienced on this planet. Were all other factors to remain constant, a return to more ice-age-like conditions would take thousands of years. However, earth's climate will probably be affected by man's activities much sooner, as it already has been. Can these activities speed or delay inevitable climatic changes or trigger events that otherwise would not have occurred? These are among the most critical questions being studied by climatologists today.
Before the industrial revolution, man's impact on the global climate was probably insignificant. Since the beginning of the nineteenth century, however, vast areas of the earth's surface have been modified. The forests of Europe and eastern North America have largely disappeared, and the grasslands of the central United States and Canada have been converted to crop production. Dams have been built and large lakes created. Swamps have been drained. Some semiarid areas have become deserts, and some deserts have been greened by irrigation. All of these changes have altered the surface reflectivity for solar radiation, water balance, temperature, and roughness of the earth's surfaces. Such alterations of surface conditions alter the climate of the affected areas and of areas downwind. Analyses are not yet available of the net, overall effects of these changes in land use on global climate, although techniques are being developed for evaluating their influence at the regional scale, and complex mathematical models of global climatic processes are now being used for this purpose.
Some changes in land use—the clearing of forests and plowing of grassland sod—have also caused large quantities of carbon dioxide and other gases to be released to the atmosphere. Drainage of swamps has probably reduced the quantities of methane emitted into the atmosphere, while the extension of rice paddy culture has likely had the opposite effect. Heavy use of nitrogen fertilizers is seen as a source of the increasing nitrous oxide content in the atmosphere. Other emittants created by industrial combustion processes and space heating have increased the content of ozone in the lower atmosphere. All of the trace gases—carbon dioxide, methane, niacin oxide, and ozone—contribute to the greenhouse effect. One other category of gases—the Freons (chlorofluorocarbons)—also contribute to this effect and, in addition, increase the concentration of ozone in the troposphere.
The combustion of fossil fuel for power generation and space heating, the operation of nuclear reactors, and many industrial processes convert large quantities of energy into heat which is released directly into the environment. It has been suggested that by some time late in the twenty-first century, heat released to the atmosphere by man's activities might equal 1 percent of the solar energy absorbed by the earth. Even today, however, all the energy consumed by humanity is less than one ten-thousandth of the solar energy absorbed by the earth.
Is climate really changing?
Temperature and precipitation are the parameters of weather that have been measured most widely and for which there are records of longest duration. Of these, the temperature records are the most likely to provide evidence of climatic trends or changes, since a thermometer represents a wider area than does a rain gauge. Precipitation is notoriously variable over short distances, especially in regions where much a the rain falls from scattered cumulus clouds.
Evidence of long-term trends in hemispheric and global mean temperature shows that a general worldwide warming trend has occurred over the past century. The data summarized in figure 1 (Resources, Winter 1987, p. 2) provide the strongest evidence yet assembled in support of this view. These data also show that the warmest three years on record have occurred in the 1980s. The global temperature trend is also repeated in records for the United states assembled by the National Climatic data Center. These records show warming until 1940 followed by cooling until the early 1960s and a return to warming.
Reliable data for global trends in mean precipitation are more difficult to come by. A long-term drought in the Sahel, the region of Africa south of the Sahara, has persisted so long as to suggest that a true climatic change may be occurring there. An analysis of rainfall records for Africa, shown in figure 3, supports the contention of an unusually long run of dry years in the Sahel since the late 1960s and of wetter conditions from 1950 to the onset of the drought. Yet the recent drought is not unprecedented in its severity or duration, and it, too, may not yet be taken as evidence of a true climate change.
The preceding discussion relates to changes in the mean or average climate. However, evidence for a consistent global pattern of change in climatic variability is Less clear. Theoretically, a global cooling, by increasing the temperature difference between the equator and the poles, should lead to increased circulation in the polar vortex. Principal pathways of cyclones shift southward with cooling and northward with global warming, and both cooling and warming tend to occur to a greater absolute extent in the polar regions than in the equatorial regions. Waves in the vortex are amplified and weather extremes become more frequent. As has been pointed out by climatologist V. Y. Sergin, more intense atmospheric circulation and shifting of the zone of highest wind intensity southward take place as the temperature gradients become larger (i.e., climatic cooling is occurring); changes in the opposite direction take place with warming.
Cool periods in history appear to be periods of enhanced instability of climate characterized by extremes such as droughts, floods, excessively hot summers, and excessively cold winters. Yet there is no clear evidence for an increase in temperature variability during recent decades and no clear linkage between global temperature and variability. Nor is there clear evidence of significant changes in the variability of precipitation throughout the world. A greater agricultural sensitivity to severe climatic anomalies such as interruptions in monsoonal rainfall in India and sub-Saharan Africa may be misinterpreted as indicating a changing variability in precipitation. A much finer network of rain gauges distributed worldwide and including coverage of the oceans will be needed before reliable statements can be made concerning changing variability in precipitation.
Agriculture—a sensitive indicator
Agriculture is the human enterprise most sensitive to change in general climatic conditions and in climatic variability. Increases in variability of annual yields of major U.S. grain crops since the early 1970s have been documented. Some attribute these trends to an increase in climatic variability, or, possibly, to a return after the early 1970s of a more normal degree of variability following an abnormally quiet period in the 1950s and 1960s.
However, other factors, including the genetic uniformity of seeds. and increased mechanization, that permit nearly simultaneous planting of crops over wide regions seem more probable causes in that they increase vulnerability to the detrimental impacts of large-scale weather systems. Additionally, plant breeding and improved agronomy have probably reduced downside variability in cereal yield but may have increased upside swings. Since yields are far greater now than they were thirty or forty years ago, bad years can be expected to cause a wider absolute, but not relative, reduction in crop yields. The increasing variability in crop yields cannot be attributed wholly to increasing variability in climate.
In summary, a long-term trend toward higher temperature beginning about 100 years ago has been observed. While significant changes in rainfall may have occurred or may not be occurring in certain places in the world, there is no evidence that these changes are unprecedented and not part of the normal variation to be expected in regional climatic conditions. Had we a better record of precipitation throughout the world it might be possible to detect some clear trends. However, because of the spatial inadequacy in the distribution of weather and precipitation sensors, no clear-cut global patterns of changing precipitation can be discerned.
As for changing climatic variability, here too the record will not sustain a global generalization. For each region in which an increase in temperature variability has been identified, another with decreasing variability or no change can be found. The available evidence does not confirm the existence of any global trend toward either increasing or decreasing climatic variability.
A study by climatologist T. R. Karl concludes that ample evidence suggests that many areas in North America have had statistically and practically significant changes in mean temperature for certain months, seasons, and on an annual basis; but it is more difficult to show statistically significant change in the precipitation climate apparently because of the greater natural variation in precipitation over time and space. Furthermore, the lack of a demonstrated statistical significance in precipitation variability does not mean that these changes are less important than those in temperature—since in North America (and many other regions in the world) water may be of greater importance than temperature.
What's coming next?
What can climatology tell us about the coming decades? The climates of the past offer insights as to what might happen in the future. Additionally, mathematical models that permit realistic simulation of processes that occur in the atmosphere and in its interactions with land, ocean, and cryosphere (snow and land and sea ice) are now becoming available. Although not yet perfect, the Global Climatic Models (GCMs) are considered the best for predicting climate in the near future. A review of their findings is worthwhile.
GCMs have been used to predict the impact of a continued increase in atmospheric carbon dioxide concentrations. Results of a number of GCMs are in close agreement in predicting a change in global average surface temperature of 1.2° C to 1.3° C resulting from a doubling of carbon dioxide concentration if feedback processes (changing cloudiness and oceanic capture or release of heat) are not considered. When these feedback processes are considered, however, the models predict that there will be greenhouse warming of the global average surface air temperature of about 3.5° C to 4.2° C and an increase in the global average precipitation rate of about 7 to 11 percent. The models agree closely with respect to global average surface temperature but much less well in their projections of the regional patterns of such changes.
Equilibrium changes in surface air temperature are predicted to be greatest in the high latitudes near the snow and ice boundaries. Reductions in the extent of snow and ice, however, mean that the predicted zonal warming will be greatest in the winter half of the year and least in the summer half in the high latitudes.
The models also predict greatest changes in the distribution of precipitation in the belt between 30° N and 30° S. Increased precipitation is predicted in this zone, with decreases in the adjacent zones at least during parts of the year. A number of the GCMs predict that the grain belts of Northern Hemisphere continents will become drier especially in the spring and summer. The various models disagree more in their predictions of precipitation change than in their predictions of temperature change.
What do the next decades hold? Obviously there can be no unequivocal answer. There is a broad consensus among climatologists and other involved scientists that, if no unexpected changes in solar luminosity occur and if volcanoes are not extraordinarily active, the greenhouse effect caused by carbon dioxide and other radiatively active trace gases will become indisputably detectable in the next decades. The world will be a wanner place than we have known, and precipitation patterns will be different in most regions than they are today. Changing temperature and precipitation, in combination, will profoundly affect production agriculture, forestry, and water resources throughout the world.

Norman J. Rosenberg, formerly George Holmes Professor of Agricultural Meteorology at the University of Nebraska, is a senior fellow in RFFs Renewable Resources Division and director of its Climate Resources Program.