Whatever goes up, must come down. But does it? And if so, where, and in what form and concentration?
Acid deposition—acid rain is only one form—seems easily understood at first glance: gases of sulfur and nitrogen are released into the air, where portions of them are converted to acids that fall to ground in rain, snow, or as dry deposits on surfaces. Since some lakes, trees, and other biological species apparently are adversely affected by the acids, it is desirable to reduce the amount deposited.
But how can or should this be done? Logic suggests reducing the amounts of sulfur and nitrogen released into the atmosphere. In the northeastern quadrant of the United States, where the problem is greatest, most of the sulfur dioxide and about half of the nitric oxide are released by power plants burning oil and coal. The most obvious solution—forcing such plants to reduce their emissions of one or both of these compounds—would require for most the use of sulfur dioxide scrubbers at costs that could total $100 billion during the remainder of this century. Many laymen have a "sock-it-to-them" attitude toward the power companies, but ultimately utility customers would pay the bill. Thus, solutions to the problem affect a large segment of the population, and not only power company shareholders.
What is acid rain?
An acid is a substance that releases hydrogen ions in water solution. Chemists use "pH" to describe the relative acidity or alkalinity of a solution, and this term has been picked up by the popular press. This often is misleading because, as the negative logarithm of acid concentration, pH varies much less than the concentration itself and, for acids, in the opposite direction of acidity. To a layman, the difference between pH5 and pH4 appears to be only a 20 percent change, and pH4 sounds better than pH5, but in reality a solution with pH4 contains ten times as much acid as one of pH5. In this article I use the actual hydrogen ion concentrations in terms of microequivalents (Reg) per liter. For example, pH4 is 100 μeq per liter and pH5 is 10 μeq per liter.
All water contains some acid. In pure water a few water molecules break up into hydrogen and hydroxide ions, so the acid concentration is 0.1 μeq per liter. Pure water in the natural, unpolluted atmosphere contains additional acid because carbon dioxide from the air dissolves to form weak carbonic acid, producing a hydrogen ion concentration of about 3 μeq per liter. In addition, the atmosphere contains natural sulfur dioxide, resulting from biological activity in the oceans and on land, with some of it being transformed into sulfuric acid. The amount of acid from natural sources is not well known, but it is rarely more than 10 µeq per liter. Thus, rain naturally is somewhat acidic, but human activities clearly have made it more so: For example, in the eastern United States, the acid concentration in individual rainfall events ranges from 50 to 200 μeq per liter or five- to 20-fold larger than natural concentrations.
Drops in the bucket
Since the mid-1960s, acid rain has been of serious concern in Scandinavia, especially Sweden, but it received little attention in the United States until the mid-1970s, when Gene Likens and his co-workers, then at Cornell University, published maps of rainfall acidity in the Northeast showing that acidity had increased substantially between the mid-1950s and the mid-1970s. These maps, coupled with reports of "dying lakes" in areas such as upper New York State and Canada, greatly increased public awareness of this possibly growing problem. However, careful examination of the data raises serious doubts about their reliability, especially those gathered in the 1950s.
Accurate measurements of rainfall acidity are difficult to perform. For example, if a bucket is placed in a field and the rain collected in it is analyzed once each week, the results can be wrong for many reasons. One is that particles suspended in the atmosphere that contain alkaline substances may fall into the bucket and neutralize the acids, thus producing acid concentrations that are too low. Until recently, reliable techniques had not been established for measuring the acidity of rainwater, so that the older data cannot be corrected for problems associated with their measurement.
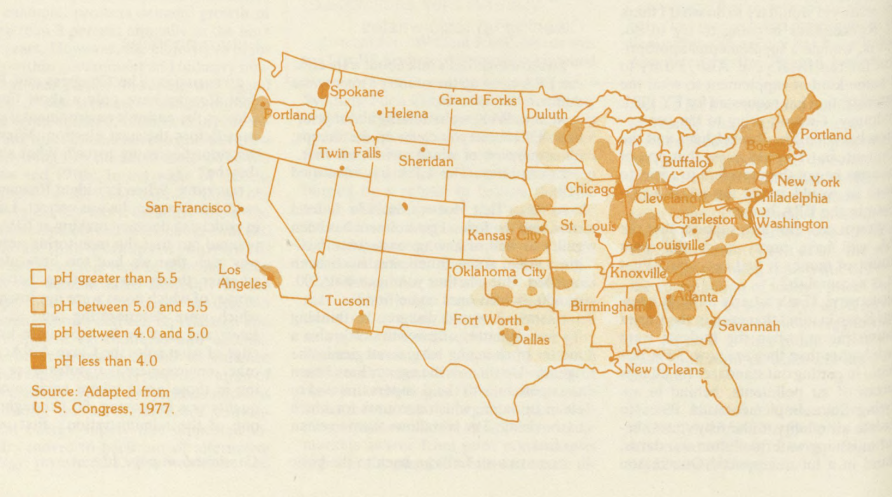
The longest continuous record of rainfall acidity is that obtained at Hubbard Brook Experimental Forest, New Hampshire, beginning in 1964. Between 1964 and 1980, the annual average acidity ranged between 60 and 95 μeq per liter and, if there is a long-term trend, it is slightly downward. Even when data for acidity (or concentrations of most other pollutants) are averaged over twelve months, year-to-year fluctuations are so great that data must be obtained for many years to establish a clear trend. Since 1964, sulfate concentrations in precipitation at Hubbard Brook have declined by about 20 percent, possibly reflecting reduced emissions of sulfur dioxide in the East. Nitrate concentrations rose from 1964 to 1971 (probably because of increased emissions of nitrogen oxides by motor vehicles) and have been fairly constant since then. Thus, the Hubbard Brook record of acid precipitation suggests that acidity has remained constant, or has declined slightly, since 1964, and that the reduction in sulfuric acid has been balanced approximately by increased nitric acid, with the former now accounting for about two-thirds of the acid.
Producing acid rain
Whether or not rainfall acidity is increasing, considerable political pressure is being exerted, especially from Canada, New England, and New York, for its reduction. The classic scenario resulting from many studies is that air masses move up the Mississippi and Ohio River valleys, collecting emissions from many power plants, especially those along the Ohio River. During transit, the air pollutants slowly form acids and sulfate particles, causing acid precipitation in that area and to the northeast. Indeed, maps of acid precipitation show that the region of high acidity extends considerably farther toward the Northeast than does the area of high-density sulfur dioxide sources. Despite the apparent strength of this argument, two major questions must be answered before it is clear that reducing emissions will be beneficial: Will a given reduction of sulfur and nitrogen oxide emissions produce a proportionate reduction of acid? And if reductions are made in a given area, at what distance will there be a significant effect on precipitation acidity?
Oxidizing agents
These questions can be answered only when the major mechanism(s) that produce acid precipitation are fully understood or when empirical studies demonstrate the relationship between release of pollutants at one point and deposition of acids at another. Production of acid requires at least two reactants: the sulfur or nitrogen oxide gas and an oxidizing agent that transforms them into sulfuric and nitric acids. Until the most important oxidizing agents are established, it is impossible to know which species is in excess. If, for example, it is sulfur dioxide, then the amount of sulfuric acid formed will be limited by the amount of oxidant available. If that were the case, emissions of sulfur dioxide would have to be reduced to the point at which its concentration was equal to the available oxidant before further reductions would decrease the acidity of precipitation. This is a definite possibility, as it is fairly certain that much of the sulfur dioxide released in the Northeast is not deposited within the area. Of the estimated 8 million metric tons of sulfur released there, about 3 million metric tons are transported to the Atlantic Ocean without being deposited and another 1 million tons go to Canada. Thus, additional sulfur could be deposited in the area, but luckily it is not. Is that because there is a shortage of the oxidizing agent?
Although present knowledge of the atmospheric chemistry of sulfur and nitrogen oxides is far from complete, progress in research has been rapid during the past several years.
Photochemical reactions
It is known, for example, that during the summer, some sulfur dioxide is oxidized by a hydroxyl radical, a highly reactive, transient species produced by reactions caused by sunlight, that is, photochemical reactions. Because hydroxyl radicals react so quickly, their concentrations are never very large and, as a consequence, no reliable, direct measurements have been made. Reactions of hydroxyl radicals may produce much of the sulfate borne by atmospheric particles, which causes the haze that blankets the Midwest and East, especially during summer. But even if they are all brought down by rain, these particles do not carry enough acid to account for the acidity of the rainfall. Other photochemical reactions convert nitric oxide to nitric acid, which is a gas unless water droplets are present. If this is the case, most of it dissolves. But even if the nitric acid below the clouds also is collected by the falling rain, the total acid is not enough to produce such an effect. Apparently, much of the acid is produced in clouds.
The role of clouds
Clouds provide a good medium for chemical reactions, as gases can dissolve in cloud droplets and react with each other. Dissolved oxygen and sulfur dioxide can form sulfuric acid, but the reaction is too slow in pure water to account for the amount of acid that has been observed. The reaction can be speeded up (catalyzed) by dissolved metals such as iron and manganese, but the process slows down after some acid has been formed.
One important oxidizing agent in cloud droplets is hydrogen peroxide, which is produced by photochemical reactions of nitrogen oxides and hydrocarbons. Unlike other reactants, hydrogen peroxide keeps on reacting after some acid has been produced. However, hydrogen peroxide is far from being established as the most important oxidant. Only recently has it been possible to measure its concentrations in the droplets of very calm, non-raining clouds.
Another possible oxidant in the cloud droplets is ozone, yet another product of photochemical reactions. Concentrations of both sulfate particles in the air and acid in rainfall are higher in summertime, when the intensity of the light is greatest, despite the fact that the concentration of sulfur dioxide is higher in the winter and nitrogen oxides are fairly constant throughout the year. Some acid and sulfate are produced in winter, which leads one to believe that other reactions may be important as well, for example, catalysis by manganese and iron.
Clouds play an important role in the production of acid, but aspects of their performance remain poorly understood. Most people are not aware that few clouds produce rain. Instead, they evaporate, leaving behind the solid material that was present in the droplets. Such a particle released into the air passes through about ten cloud droplets before it is deposited at ground level; the average suspended particle has been through five clouds. Measurements taken in clouds show that they contain much more acid than do the residual particles. What happens to the acid between clouds? Part of the answer is that the nitric acid gas dissolved in cloud droplets evaporates along with the water. However, this does not explain why more sulfuric acid is not found on the particles. Perhaps this dilemma results from poor knowledge of bases, the acid-neutralizers. Much of the particulate matter in the atmosphere is soil and rock dust containing basic substances, such as limestone, that neutralize some of the acid. However, an even more important base is ammonia gas, released by feedlots, fertilized fields, and biological processes. The concentration of ammonia gas probably never gets very large because it reacts rapidly with sulfuric acid particles to neutralize them.
According to this picture, acid is made in clouds that evaporate, but much of the acid formed is neutralized by ammonia while the particles are between clouds. In my view, the majority of acid is made in the clouds that produce rain. In these clouds—the more active of which cannot be sampled safely by aircraft—huge up-drafts suck in large volumes of air at their bases. The turbulence promotes mixing of various species, giving them an opportunity to react in the cloud droplets. Such clouds may make acid so rapidly that it cannot be neutralized by ammonia gas before it falls to earth in rain or snow.
This scenario, based on clues gleaned from studies of air and gentle clouds, is speculative, but the enormous progress being made in atmospheric research should provide fairly clear knowledge of the important pathways for acid formation and neutralization within the next five years. Measurement methods for some key species, available only in the last year or so, already are providing vital new information.
Report of the National Research Council
Last year, a group of distinguished atmospheric scientists, brought together by the National Research Council, reviewed all available information and arrived at the following carefully worded conclusion:
"If we assume that all other factors, including meteorology, remain unchanged, the annual average concentration of sulfate in precipitation at a given site should be reduced in proportion to a reduction in SO2 and sulfate transported to that site from a source or region of sources. If ambient concentrations of NOx, nonmethane hydrocarbons, and basic substances (such as ammonia and calcium carbonate) remain unchanged, a reduction in sulfate deposition will result in at least as great a reduction in the deposition of hydrogen ions."
Although I respect the opinion of the panel, I am not as confident that reducing sulfur oxide emissions by X amount would yield a proportional reduction in acid deposition. If the acid is produced in clouds that rain, those clouds may exhaust their oxidants before they convert all the sulfur dioxide into acid. If so, a small reduction in sulfur dioxide emissions might produce no observable change in acid deposition, especially in view of the great year-to-year variability of average acidity.
The panel's report indicates that it is impossible to predict the distance at which rainfall acidity would be affected by reducing sulfur dioxide emissions in a particular locale. As noted above, the area of highly acid rainfall extends considerably farther toward the Northeast than the area of high sulfur dioxide emissions of the Ohio River Valley, empirically suggesting that, for long-term averages, the effects probably would extend several hundred miles. However, in my view, if one considers a particular mass of air traveling from the Ohio River Valley to the Northeast, the area of acid deposition can be determined largely by the pattern of rainfall along its path. If rain occurs near the midwestern sources, then little acid will be carried to New York and New England. But if no rain occurs in the air mass until it reaches the Northeast, then acid in that area will result in part from emissions released in the Midwest.
Two other points should be noted. First, I have focused on the deposition of acid, especially sulfuric acid. Acids, of course, attack a wide variety of materials. However, many experts on biological effects are more concerned about total sulfate deposition, as some biological processes convert sulfate of any type of sulfuric acid. Second, only recently have scientists begun to study fogs, whose droplets usually contain much greater concentrations of acid and sulfate than raindrops—and the fog droplets are deposited directly on the leaves of vegetation. Much damage to trees and other vegetation in areas of frequent fogs may be caused by acid fog rather than rain.
Reducing emissions
Some proponents of strong measures to control sulfur oxide emissions note that many past decisions, though based on incomplete information, have been successful. One, they point to the control of coal burning in London after thousands died as a result of the great London fog of 1952. But other similar decisions probably have been ineffective. For example, no convincing case has been made for the benefits (outside of southern California) of reducing carbon monoxide and hydro-carbon emissions from automobiles by requiring catalytic converters. (Indeed, the major benefit may have been a great reduction in atmospheric lead: leaded gasoline cannot be used in vehicles equipped with converters.)
Congress currently is considering several bills calling for reduction of sulfur oxide emissions, primarily by the electric power industry. Not surprisingly, states with the greatest emission densities favor regulations that would require uniform percentage rollbacks, while those with low emissions favor a reduction by states with high emissions. It is unfortunate that this decision will be based on regional political considerations rather than on maximizing environmental benefits while minimizing costs. Certain areas are much more sensitive to acid rain than others, in part because their soil and rocks do not have much capacity for neutralizing acid. As some of the areas of greatest sensitivity are in upper New York State, New England, and eastern Canada, any plan to reduce sulfur oxide emission should focus there.
One probable side benefit of reducing sulfur dioxide—clearer skies—has received little attention. Even if the reduction of acidity of rainfall is not proportional to the reduction of sulfur dioxide emissions, there is a good chance that concentrations of sulfate particles in the atmosphere will decrease. And as sulfate particles cause most light scattering in the atmosphere, significantly fewer of them should mean reduced haze and improved visibility.

Author Glen E. Gordon is professor of chemistry at the University of Maryland, He was a Natural Science Fellow at RFF during 1982-83.